Part 1 of this article last month covered directed energy weapons, kinetic energy weapons and particle beam weapons. This part covers the involved technology areas and advantages as well as limitations of the particle beam weapons.
Different technology disciplines related to development of particle beam weapons include particle accelerator, power source driving the accelerator, target-tracking and beam-pointing system, beam propagation through medium separating the source and the target and lethality (Fig. 6).
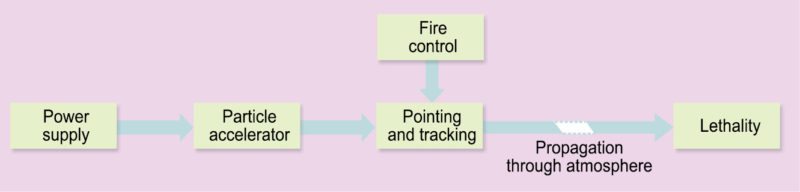
Particle accelerator technology
The particle accelerator is the source of high-energy particle beam and is therefore the heart of the particle beam weapon system. Three main constituents of the particle accelerator are: the source of particles such as electrons, protons or charged atoms, the device that injects these particles into the accelerating section and finally the accelerating section itself.
The particle accelerator accelerates particles to extremely high energies. These particles, as outlined earlier, are elementary particles like electrons or protons, or even whole atoms. In modern accelerators the particles very quickly attain nearly the speed of light, which is about 3×105 km/s. According to Einstein’s theory of relativity, this speed can only be reached by massless particles and can never be exceeded by any particle. Though the speed of a particle cannot be increased any further after it has attained near speed of light, its kinetic energy can still be increased. All particle accelerators accelerate the particles roughly to the speed of light; difference between more or less powerful accelerators is the kinetic energy of the accelerated particles.
There are two types of accelerators, namely, the linear accelerators and the circular accelerators (also called ring accelerators). Linear accelerators accelerate particles over a long, straight line, where the particle beam travels from one end to the other. In circular accelerators, powerful magnets are used to bend the particle’s path into a circle and the beam of particles travels repeatedly in a loop. Technologically, both linear and circular accelerators are in a highly advanced state of development. The preferred accelerator technology depends upon the application.
In earlier accelerators, strong electric fields were used to accelerate the particles, but the maximum usable electric field strength had an upper limit due to spontaneous discharge. The accelerating section of all conventional linear accelerators therefore is made up of a cascade arrangement of a number of accelerating segments that sequentially apply an accelerating electric field to the charged particles. While the voltage in each segment may be relatively low, the repeated application of an accelerating voltage by the large number of modules ultimately produces substantially high particle energies.
Modern accelerators use focusing magnets to ensure that particles traveling at the speed of light are not lost and always remain on their reference trajectory. SLAC’s 3.22km long particle accelerator (Fig. 7) is an example of a linear accelerator. Circular accelerators employ strong bending magnets to keep the particles on their circular path. Smaller the diameter of the circular path, larger and more powerful the focusing magnet needs to be.
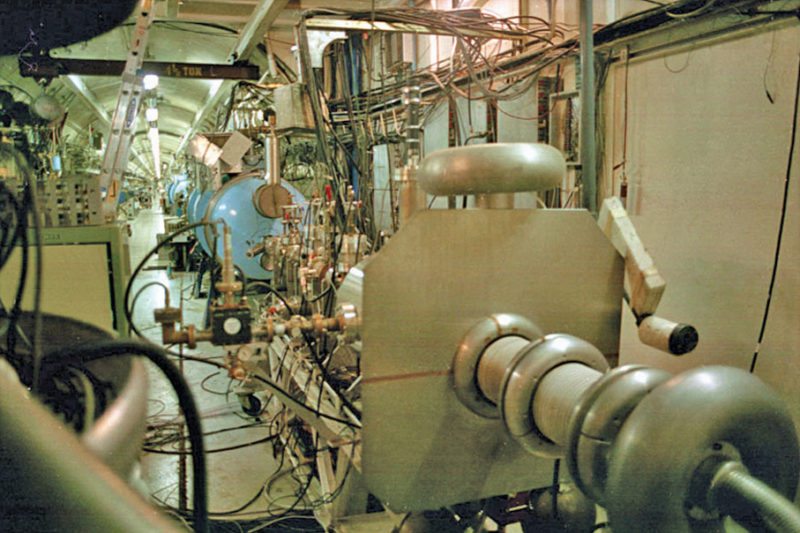
The Large Hadron Collider (LHC) is an example of a ring accelerator (Fig. 8). It is the world’s largest and most powerful particle accelerator. The LHC has a 27km ring of superconducting magnets with a number of accelerating structures to boost the energy of the particles along the way. Inside the accelerator, two high-energy particle beams travel in opposite directions in separate beam pipes at ultra-high vacuum, very close to the speed of light, before these are made to collide.
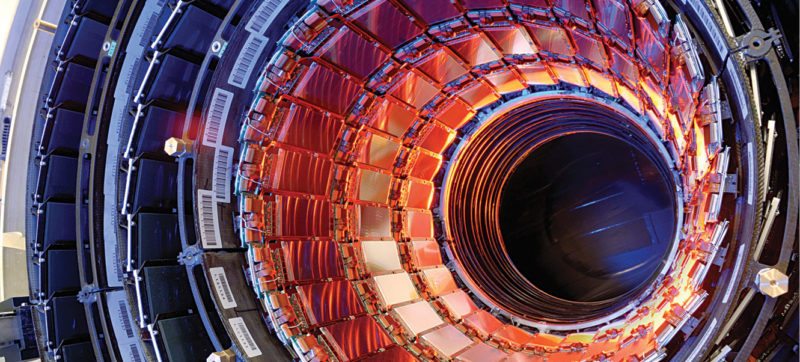
Two common types of linear accelerator technologies used for development of particle weapons include RF linear accelerators (RF LINACS) and induction linear accelerators (induction LINACS). Although linear accelerators are capable of accelerating particles to energy levels high enough for use as a weapon, their current-carrying ability is severely limited. As a consequence, these are not suitable for building accelerators for endoatmospheric particle beam weapons. RF LINAC can be a suitable candidate for building accelerators for exoatmospheric particle beam weapons as space weapons don’t call for very high beam power.
Both RF LINAC and induction LINAC use successively high voltages across a series of accelerating segments. Induction LINAC differs from RF LINAC in the mechanism used for generating the electric voltages within the segments of the two types of LINACS. Compared to the RF LINAC, the induction LINAC produces a more stable beam at high beam currents. The induction LINAC is therefore a more likely candidate for an endoatmospheric beam weapon.
Power source
Development of power source for an endoatmospheric particle beam weapon is a big technological challenge. The power supply is required to supply high energy over short time periods, which translates into very-high-pulsed power levels. Advanced pulsed power technology is therefore required to develop power supply for a particle beam weapon.
A pulsed-power device comprises three main parts: the prime power source that provides the required electrical energy over the full operating time of the weapon, energy-storage device needed for intermediate storage of the electrical energy as it is generated, and the pulse-forming network that generates power bursts or pulses of desired magnitude and time duration. Each of these three areas presents a major technological challenge.
The prime power source of a particle beam weapon is required to deliver power levels in the range of mega-watts to giga-watts, and at the same time be as lightweight and compact as possible. Also, in many applications, the prime power source needs to be mobile. Though a conventional power station could provide the needed power levels, it would be neither small nor lightweight, and would definitely not meet the mobility requirement. Some of the potential candidates for building the prime power source include advanced technology batteries, turbo-generators and advanced magneto-hydrodynamic (MHD) generators using superconducting circuitry.
A typical energy-storage method involves charging a bank of capacitors, or spinning a huge mechanical flywheel, or simply storing the energy in the form of a high-energy explosive that is released in a contained explosion. There are a number of other energy-storage and release mechanisms, each having its own set of advantages and disadvantages. The preferred mechanism depends upon the particle accelerator and also whether the beam weapon is endoatmospheric or exoatmospheric.
The pulse-forming network is used to shape the power pulse. In the case of an endoatmospheric particle beam weapon, one shot would have a burst of very-short-duration pulses with burst frequency in the range of kHz. The prime power source may deliver power for a series of bursts of pulsed power followed by the beam weapon going to quiescent state while the energy storage device gets recharged for another series of bursts of pulsed power.
Target tracking and beam pointing
Unlike some other areas of particle beam weapon development such as the generation of high-energy particle beam, which need to address certain basic issues of science and technology, target acquisition and tracking and beam-pointing technologies required for a particle beam weapon are not unique to this class of directed-energy weapons. In this respect, particle beam weapon programme has immensely benefitted from high-energy laser weapon programme.
Notwithstanding commonality of technologies between the particle beam weapons and high-energy laser weapons, there do remain some tracking and pointing problems that need to be solved. Many of these arise out of propagation of charged-particle beam through the atmosphere and neutral-particle beam through space.
Beam propagation
Other than the high-energy particle beam source and target-tracking and beam-pointing technologies, third important element determining the success of a particle beam weapon is the propagation of the charged-particle beam through atmosphere in the case of endoatmospheric weapons and neutral-particle beam through space in the case of exoatmospheric weapons. The particle beam must have an extremely precise path of propagation as it traverses the required distance to the target.
The propagation problem is more severe in the case of charged-particle beam through atmosphere as compared to that of neutral-particle beam through space. Propagation of a neutral-particle beam does not suffer from beam instability problems possibly encountered by a charged-particle beam while propagating through atmosphere.
One of the contributing factors is beam spreading, which causes increase in beam diameter and consequent decrease in energy density as it travels towards the target. Even with a small amount of beam divergence, the beam diameter may become appreciable enough to be unacceptable for longer ranges.
Two factors contribute to beam spreading: beam divergence imparted by the accelerator itself and present at the exit of the accelerator, and mutual repulsion of beam particles. While in the case of charged-particle beams both the factors are responsible for beam spreading, it would strictly originate from accelerator in the case of neutral-particle beams.
Even in the case of a neutral-particle beam propagating through atmosphere, the surrounding air molecules strip the neutral particles of the electrons and transform it into a charged-particle beam. This would further lead to undesirable beam divergence
In the atmosphere, however, even if the beam particles were neutral, air molecules would strip the surrounding electrons quickly from the beam’s neutral atoms, turning the beam into a charged-particle beam. The charged particles within the beam would then tend to repel one another, producing undesirable beam divergence. The unwanted effect is countered to some extent as the propagating beam may knock off electrons from air molecules, which would in turn intermingle with the beam and neutralise the charged particles. The magnetic field created by the charged-particle beam current also prevents beam spreading by producing a type of conduit. For a charged-particle beam to propagate satisfactorily through the atmosphere, the particle beam needs to have certain threshold values of beam parameters including beam current, particle energy and pulse duration.
The problem is still looking for a solution as currently no particle-beam accelerator is capable of producing a beam with the required parameters. Two important experimental programmes with particle accelerator are exploring the phenomenon of particle-beam propagation through atmosphere. One of these relates to the experiments with Advanced Test Accelerator (ATA) at Lawrence Livermore National Laboratory and the other is a joint Air Force/Sandia National Laboratories programme through the use of Radial-pulse-line Accelerator (RADLAC).
Lethality
Lethality studies play an important role in understanding the precise effect a particle beam would have on interaction with different types of target materials. The subject becomes further complex as the particle beam-matter interaction would depend upon type of particles in the beam, particle energy and beam power. Parameters determining lethality and hence the efficacy of the beam weapon include beam velocity, dwell time, rapid retargeting capability, beam penetration, ancillary kill mechanisms and all-weather capability.
A beam velocity that is much higher than the target speed simplifies the task of fixing the aim point even in the case of an evasive target. As an example, a target at a distance of 50km and moving at a supersonic speed of 6 Mach would have moved only a metre or so from the time the particle beam weapon is fired till it hits the target. This is made possible by the particle beam travelling at near speed of light of 3×105 km/s. The beam dwell time—the time period for which the particle beam is needed to remain on the aim point on the target to inflict intended damage—is of the order of a few microseconds in the case of endoatmospheric weapons. In this case, beam power is sufficient enough to cause almost instantaneous damage. Short dwell times may be required in case of exoatmospheric beam weapons due to comparatively lesser power of these weapons.
Rapid retargeting feature of the particle-beam weapons gives them the capability of engaging multiple targets. This is made possible as the charged-particle beam can be deflected in the desired direction, within certain limits, with the help of a changing magnetic field. Unlike high-energy laser weapons, where the laser-matter interaction effects are mainly confined to the surface, particle beams have much greater penetration. The structural damage in this case is far more severe, sometimes leading even to a catastrophic damage.
Also, in the case of laser-induced damage, the material blowing off from the target surface tends to envelop or hide the target, thereby acting as a protective shield. Particle-beam weapons with their penetrating nature do not suffer from this problem. Even target hardening through shielding materials selection proves to be ineffective.
Particle-beam weapons offer many ancillary kill mechanisms, which are capable of inflicting sufficient damage to the target even if the main beam misses the aim point. One of the ancillary kill mechanisms is the presence of a secondary cone of radiation symmetrical about the main particle beam. This radiation cone comprises X-rays, neutrons, alpha- and beta-particles etc, and is created by collision of beam particles with atoms of the air.
Another ancillary mechanism is electromagnetic pulse (EMP) generated by the presence of beam-current pulse. The EMP could play havoc with the electronic components and devices of the target. As these ancillary mechanisms are present due to interaction of charged particles, these are not present in the case of neutral-particle-beam based exoatmospheric weapons. Poor weather conditions such as presence of clouds, fog and rain severely limit the capabilities of high-energy laser weapons. Particle beam weapons have all-weather capability.
Advantages and limitations
Major advantages of particle beam weapons include speed-of-light delivery, rapid re-targeting, all-weather capability, better target interaction and shorter dwell time.
Speed-of-light delivery allows particle beam weapons to precisely target even fast-moving and evasive targets, which is a big advantage in anti-ballistic missile (ABM) defence. It also enables precise aim point selection on fast-moving targets at longer ranges.
Rapid re-targeting is enabled by use of changing magnetic field to deflect the charged-particle beam within certain limits without any requirement of physical movement. This feature is not available in neutral-particle beam weapons.
Particle beam weapons have all-weather capability and, unlike high-energy laser weapons, their use is not limited by atmospheric factors such as rain, fog, clouds, dust, etc.
Particle beam weapons—due to their high particle energy coupled with secondary and tertiary kill mechanisms in the form of cone of radiation of X-rays, alpha- and beta-particles etc and EMP—interact much better with the target. Particle beams have much more impact damage on the target than the massless photons of the high-energy laser weapon, and the penetration increases with increase in particle energy. Presence of ancillary kill mechanisms increases manifold the probability of target damage. This makes it possible to inflict damage to the target even if the main particle beam misses the target.
Dwell-time requirement of particle beam weapons is much less as compared to that of high-energy laser weapons. In the case of charged-particle-beam based endoatmospheric weapons, due to high particle energy levels, the dwell time is almost negligible, being of the order of a few micro-seconds. In the case of neutral-particle-beam weapons for space usage, short dwell times would be required.
Major limitations of particle beam weapons include line-of-sight requirement, huge size and weight, massive power source requirement, thermal and electrostatic blooming and complicated beam control.
Particle beam weapons like high energy laser weapons are essentially line-of-sight weapons. Their efficacy is neutralised or reduced due to the presence of an object obscuring the target. Indirect fire used in artillery warfare is not feasible with line-of-sight weapons though one might think of reflectors on airborne or space-based platforms in the case of high-energy laser weapons enabling indirect fire.
Looking at the state-of-the-art in accelerator technology, the kind of size and weight requirement to achieve particle energy levels in the range of hundreds of MeV to GeV is so gigantic as to make it impractical as a weapon. The accelerators capable of generating weapon-grade particle energies need to be driven by electrical power in the range of tens to hundreds of megawatts, almost equivalent to the capacity of a power sub-station.
Another limitation of a particle beam weapon arises out of the beam spreading, called blooming. There is thermal blooming and electrostatic blooming. Due to blooming, particle energy that would otherwise be focused on the target gets spread out.
Thermal blooming is present in both charged-particle and neutral-particle beams. It occurs when particles bump into one another under the effects of thermal vibration and also when they bump into air molecules.
Electrostatic blooming occurs only in charged-particle beams and is due to mutual repulsion of the charged particles. In the case of neutral-particle beams therefore, the beam spreading is due to thermal blooming only.
Blooming leads to an increasing beam size as it travels the distance to the target, thereby decreasing the beam intensity and consequently the ability to inflict damage. In other words, it reduces operational range. It may be mentioned here that electrostatic blooming is present in charged-particle beams. Neutral-particle beams are not affected by electrostatic blooming as absence of charge means no mutual repulsion and consequent beam spreading.
Yet another problem with particle beam weapons is their complicated beam control, more so in the case of neutral-particle beam weapons for exoatmospheric applications. One of the reasons for this is deflection caused by earth’s magnetic field. The other reason, applicable to neutral-particle beams, is the difficulty in sensing deviation of the beam from intended path to apply correction if required.
Effects of particle beam weapons
Due to the form of energy propagated by a particle beam weapon, the mechanism by which it destroys a target is different from those of other types of directed-energy weapons, including high-energy laser weapons and high-power microwaves. Both charged-particle and neutral-particle beam weapons generate their destructive power by accelerating subatomic particles or atoms to near speed of light and focusing these high-energy particles into a beam whose energy is the aggregate kinetic energy of the particles. When concentrated into a beam, it can melt or fracture the material on interaction with the target. Target destruction in the case of a particle beam weapon occurs by kinetic penetration due to sub-atomic particles moving at speed of light, thermal damage and disruption of atomic bonds of the target.
Particle beam weapons, like other forms of directed-energy weapons, affect their targets through either a soft kill or hard kill, depending on conditions such as distance to target, power generated by the weapon and target hardening level. In the case of soft-kill damage, the effect of attack is to deny or degrade the operation of the target platform, or even inflict partial damage. Some examples include disrupting electronics of a guided missile, forcing it to miss the intended target, or damaging visible, infrared and microwave sensors on board the target platform. Though the soft-kill damage causes temporary loss of function, it can seriously compromise mission success. Hard kill relates to a permanent physical damage to the structure of the target.
To be continued…
Dr Anil Kumar Maini is former director, Laser Science and Technology Centre, a premier laser and optoelectronics research and development laboratory of Defence Research and Development Organisation of Ministry of Defence.
In 1967 when I designed the tri-beam system which uses a negatively-charged particle beam and a positively-charged particle beam aimed at a central negatively-charged particle beam that is the driving beam, a semi-neutral wrap would be formed to keep the beam coherent until it hits its target. I suggested to President Nixon in 1970 in a letter that there should be a satellite defense system that used my power ray to shoot down missiles and warheads. It took me until 1977 to design my injection reactor which would provide the charged particles. Several years ago I designed a hyperlight speed reactor which used my injection reactor to provide the charged particles that would be run through a series of cyclotrons so that when the particles almost come in contact with other charged particles, repulsion would push the particles beyond the speed of light. When the particles are accelerated enough, they would be merged into a single beam using my tri-beam system that I so powerful due to the increased energy mass that a laser compared to a power ray that is traveling at high hyperlight speed would be like comparing a kite with a Saturn V rocket. Hyperlight speed reactors would be used by repulsion-drive engines to allow vessels to travel to the stars and eventually beyond the borders of the universe.