Understanding of nano-scale phenomena, functional materials and soft devices has progressed to a point where substantial advances in stretchable electronics applications are becoming realistic.
Electronic devices in their various designs and capacities have already brought a revolution by influencing each and every aspect of our life. There is day-to-day dependence on technology, and the field of electronics is leading the way towards more advanced appliances.
Along with the development of electronics with various characteristics for different applications, stretchable electronics is emerging as a promising new technology for the next generation. Stretchable electronics is a technology for building electronic devices by depositing stretchable electronic circuits onto stretchable substrates, or embedding them completely in a stretchable material such as silicone or polyurethane.
Stretchable electronics, sometimes called elastronics, is a new and emerging class of electronics that is expected to enable a range of new applications, including cyber skin for robotic devices, imparting a network of sensors on a fully conformable, stretchable cyber skin; in-vivo implantable sponge-like electronics; and flesh-like devices with embedded electronic nervous systems.
Use of wearable electronics for personal consumption and long-term remote health monitoring is growing, and the key challenge is that the system must accommodate all types of bending, curved, soft and elastic surfaces of biological objects.
Flexible and stretchable electronics is achieving a great variety of applications, because the components can be compressed, twisted and made to conform to complex non-planar surfaces. Currently, wearable electronics applications have positive effects on various aspects of our daily life, leading to economic growth, and rapid development of stretchable electronic devices and related manufacturing technologies.
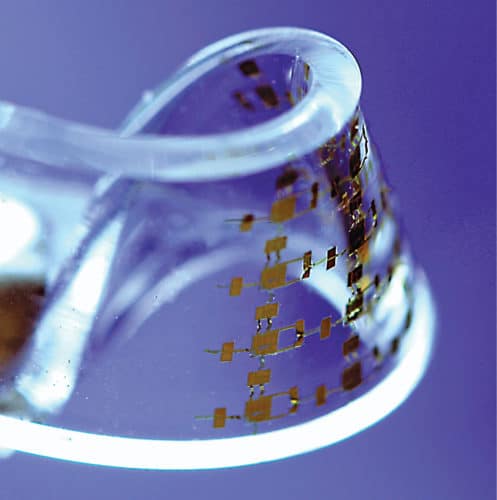
Flexible, soft and stretchable forms of electronic devices enable next-generation wearable electronic applications, opening up various avenues for healthcare, energy and military purposes. Stretchable electronics is emerging in different fields such as energy conversion and storage (including solar cells), supercapacitors, lithium-ion batteries, zinc-based batteries and nanogenerators.
Further, adding transparent properties to stretchable electronic devices will make it possible to obtain more applications, such as stretchable transparent electrodes (STEs) and transparent and stretchable electronic devices (TSEDs), where high levels of both optical transparency and stretchability are required for conformal placement of devices on the human body or any arbitrary surface.
Technological developments and challenges
The interest in fabrication strategies for stretchable electronics has been driven by the huge demand for wearable, intelligent and integrated electronic systems in the past decade. Therefore strenuous efforts have been made to realise the stretchability of electronic devices and reduce the total fabrication cost via sophisticated material design and novel device configuration.
However, mechanical compliance is critical in the manufacture of stretchable electronic devices, as devices should not incur physical damage or change their performance under bending or stretching states. A number of important discoveries and breakthroughs in the fundamentals of this technology and fabrication strategies are taking place to solve these challenges and achieve the above-mentioned targets.
Various strategies are used to make electronic devices stretchable, including directly utilising intrinsically stretchable materials or constructing structure design strategies such as wavy structure configuration, island-interconnect configuration, mesh structure, fractal design approach, origami and kirigami structural configurations.
However, stretchable conductors and electrodes are the fundamental building blocks for final stretchable electronic devices. The compliant, deformable and stretchable qualities of stretchable conductors have updated traditional ideas that people must use non-flexible silicon-based electronics. This has opened up a new direction of next-generation flexible electronics.
Development of stretchable electronic devices is not a new concept, and a wide range of stretchable electronic devices are being investigated. Further growth in such devices could have a wide range of potential uses, from wearable health monitors and elastic solar cells to artificial skin. But there are several challenges involved in finding suitable materials and manufacturing methods. The biggest challenge for making stretchable electronics is that each component must endure being compressed, twisted and applied to uneven surfaces while maintaining its performance.
Primary developing trends in flexible and stretchable electronics involve the innovation of material synthesis, mechanical design and fabrication strategies that employ soft substrates. The biggest challenge here is that the entire electronics system must allow not only bending but also stretching. Therefore stretchable conductors become a crucial construction unit for the connection of working circuits of various stretchable devices.
Owing to the success of stretchable conductors, various stretchable electronic devices are fabricated with the help of multiple manufacturing strategies, including stretchable heaters, stretchable energy conversion and storage devices, stretchable transistors, sensors and artificial skin. Continuous development of stretchable electronics has led to the new functionality of transparency, and fabrication of transparent, stretchable electronic devices has gained a lot of interest due to the potential of wearable electronic systems.
For instance, low-cost stretchable conductors and electrodes are being made from silver nanowires and graphene. Presently, an electronic thin-film transistor matrix can be stretched to about 140 per cent, and its transistor parameters also remain unchanged upon stretching.
An urgent technical problem is the need for stretchable energy conversion and storage devices such as batteries. Zinc-based batteries are promising candidates; however, more work is required to make them commercially viable. An alternative to batteries is stretchable nanogenerators, which can produce electricity from various freely available vibrations, such as wind or human body movements. Stretchable solar cells could also be used to power wearable electronic devices.
By integrating multiple stretchable components—such as temperature, pressure and electrochemical sensors—it is possible to create a material resembling human skin that could use signals from sweat, tears or saliva for real-time, non-invasive healthcare monitoring, as well as for smart prosthetics or robots with enhanced sensing capabilities. However, at present, fabrication of artificial skin remains time-consuming and complex.
Currently, there are two main strategies for manufacturing stretchable electronics. The first is to use intrinsically stretchable materials, such as rubber, which can endure large deformations. However, these materials have limitations such as high electrical resistance.
The second method is to make non-flexible materials stretchable using innovative design. For example, brittle semiconductor materials like silicon can be grown on a pre-stretched surface and then allowed to compress, creating buckling waves.
In the future, stretchable electronics may be enhanced with new capabilities such as wireless communication, self-charging or even self-healing. The next step is taking the technology of stretchable electronics from the laboratory to the market. This requires cheaper materials and faster, scalable manufacturing methods.
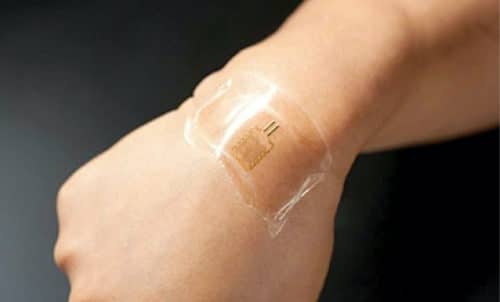
Further course of technology
Understanding of nano-scale phenomena, functional materials and soft devices has progressed to a point where substantial advances in stretchable electronics applications are becoming realistic. Materials, in general, when subjected to repeated stretch and restore process, tend to degrade in mechanical strength and recovery performance. Electronic devices worn on the body or embedded into clothing have to be made from materials that can withstand repeated use and allow no change in the mechanical properties of the device even after repeated stretching and restoration.
Panasonic Corp. has developed a polymer resin film enabling the construction of soft, flexible and stretchable electronic devices such as those developed for clothing or worn on the body. It is deployable in a broad range of applications, from wearable devices to sensors, displays and robots. This newly-developed material is an insulating film material that is said to stretch and return to its original shape—a feature that is hard to find in conventional flexible materials. It adapts to desired manners of folding and to varying free-form surfaces, substantially reducing existing design constraints. The company is also said to provide a transparent electrode material and conductive paste along with this insulating film.
Engineers at University of California San Diego, USA, have developed the first printed battery using zinc along with polymer material made from isoprene, making the battery flexible, stretchable and rechargeable. This stretchable battery can power every stretchable electronic device, from wearable sensors to solar cells and other kinds of electronics. The substance allows the battery to stretch to twice its size, in any direction, without suffering damage.
Future vision is to make 3D stretchable electronics that are as multifunctional and high performing as today’s rigid electronics. Another problem while building 3D stretchable electronics is creating electrical connections between the layers. These electrical connections, known as vertical interconnect accesses (VIAs), are essentially small conductive holes that go through different layers on a circuit. The VIAs are traditionally made using lithography and etching techniques, which work fine on rigid electronics but are questionable for their suitability on stretchable elastomers.
Presently, researchers are mixing silicone elastomer with a black organic dye so that it could absorb energy from a laser beam, forming circuits onto each layer of the elastomer. Afterwards, they are looking forward to fill in the VIAs with conductive materials to electrically connect the layers to one another. It is being reported that full rubbery integrated electronics can further be obtained by introducing metallic carbon nanotubes into a rubbery semiconductor composite from a rubbery semiconductor.
Dr S.S. Verma is professor at Department of Physics, Sant Longowal Institute of Engineering and Technology, Sangrur, Punjab.