Biomedical electronics, which combines the biological and electronics knowledge, has made appreciable technological progress in recent years. Although implantable bionic systems such as retina and cochlear implants have made remarkable advances, the potential for drug delivery applications has so far remained unrealised.
Historically, drugs were delivered by pills, ointments, injections, etc. In 1964 it was discovered that silicone rubber can be used as drug carrier for low-molecular-weight compounds in animal tissues. This concept was further developed and polymers were used as drug carriers for complex molecules like proteins, polysaccharides and polynucleotides. As a result, more complex drugs, e.g., gene-based drugs, and chimeric antibodies can be delivered to the human body. Today, synthesis and use of polymers has become the dominant approach in drug delivery system.
Generally, drugs contained in the carrier may be released by diffusion or package erosion in the digestive system. This system provides stable release rate, but the release rate is limited and enhancement of release rate requires additional chemicals, e.g., enzymes. Moreover, effectiveness of protein-based drugs can be seriously reduced by the proteases existing in the human digestive system. Although taking drugs through the digestive process may seem less efficient than via injection, it is impractical to ask patients to receive shots repeatedly or visit a clinic every day.
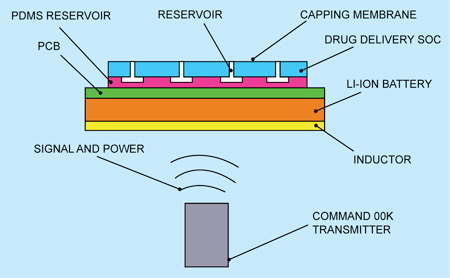
Another drug release approach is a stimuli-released system where the release of drug is triggered by specific stimuli such as electric fields, magnetic fields, enzymes, pH value and temperature change. This approach is very important for protein-based drugs. Because stimuli-controlled drug release does not depend on the dissolving procedure of the package, drugs can be preserved before the stimulus occurs. It prevents proteins from being released too early in the digestive system maintaining their efficiency. However, this system demands that the patients be treated in a hospital.
To resolve the aforesaid issues, an alternative treatment method has been developed recently. This method involves implanting a drug release system in the targeted area on the human body and triggering the drug carrier to release on demand. It envisages fabrication of a drug reservoir directly on the chip with ICs. This goal is achievable with CMOS (complementary metal-oxide semiconductor) compatible deep-trench technology. CMOS device is formed by the combination of PMOS (p-type-channel metal-oxide semiconductor device) and NMOS (n-type-channel metal-oxide semiconductor).
Working principle
A system-on-a-chip (SoC) with integrated drug reservoirs for drug delivery is shown in Fig. 1. The reservoir structure does not use an alloy as its capping membrane. Instead, it uses the passivation layer already present as a result of the standard CMOS process, which simplifies the post-IC processing procedure. The drug reservoir array is connected with the IC including a system control component and a wireless power circuit. Through these active circuits, the reservoirs may be opened by wireless command, enabling the number of reservoirs opened to be precisely controlled.
Electrolysis is used to generate microbubbles, which are employed as a force to open the reservoirs and release the drug. Wireless components, including an on/off keying (OOK) receiver, microcontroller unit, regulator, clock divider and power-on reset, are integrated for remote drug activation. The size of the microchip is about 2.48 mm2 and the power consumption is 7.57 mW.
System architecture
The architecture of the drug-delivery SoC is shown in Fig. 2. A formatted data stream is modulated by the OOK receiver and transmitted by a transmitter outside the body. The SoC implanted in the patient’s body detects the wireless signal and demodulates it using an envelope detector. Demodulation by envelope detection significantly simplifies the system architecture and reduces power consumption as it does not require any frequency translation.
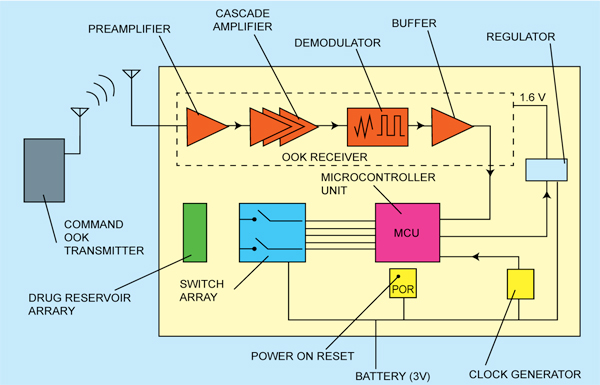
The demodulated signal is further sent to a microcontroller unit (MCU) for decoding. The decoded information contains the address of the reservoir to be opened up, so that the MCU can direct current into the electrodes of the appointed reservoir.
When the current is ‘on,’ the electrodes begin to generate microbubbles in the solution, which accumulate inside the sealed reservoir until the capping membrane is broken by the gas pressure. The power-on-reset regulator resets voltages of the MCU’s registers at the system start-up before residual voltages result in incorrect system behaviours. The regulator generates a stable supply voltage for the OOK receiver, while the clock generator is used to provide two different clocks for the MCU.
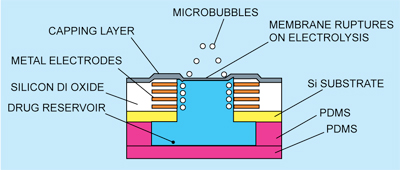
Cross-sectional view of a single unit of drug delivery system is shown in Fig. 3. The passivation layer formed by standard CMOS process is used as the membrane capping for the reservoir, which is opened up by the electrolysis approach.