Wearable devices are becoming very popular, not least as we become more aware of monitoring and improving our wellness. But for these devices to be commercial, they must be small and comfortable for the wearer while also offering advanced features and long runtimes between charging. Unfortunately, these two design requirements often conflict.
Electronic components are getting smaller and adding more features, both of which assist designers of wearable technology. However, as a result of the additional features, complexity is increasing, which can lead to a corresponding rise in power consumption. In order to retain a positive user experience, rapid charging is being employed to charge the devices quickly and advanced power management integrated circuits (PMICs) are being incorporated into wearable devices to make best use of available energy.
One of the primary applications for wearable devices is to support fitness and wellness, enabling users to monitor a range of vital signs. As the device relies on physical contact for this, where it is worn by the user has a significant on what can be measured. Most often, these devices are worn on the wrist like wristwatches, as this is a good place for monitoring most vital signs, and it is also easy and convenient for the user to access to check the results.
Users want high-performance designs that are also compact and sleek. This gives designers the challenge of coming up with ultra-low-power devices that can offer acceptable runtimes from as small a battery as possible. Short battery lifetimes therefore remain a common frustration of wearable device users.
Designing for Efficient Operation
Whatever battery size is selected for a wearable design, design teams must address the reality that there is a finite amount of energy available between charges, and design accordingly. Wearable devices are fairly complex systems, encompassing multimedia displays and a variety of sensing technologies. As a result, these devices incorporate a variety of analog and digital circuit elements, which are often segmented into domains that can be activated and deactivated as required.
The exact architecture of a wearable device will depend on the device itself, but will often include a microcontroller, memory, communications, user display, sensors and power management. The power management is usually based on a PMIC that handles the charging and incorporates DC-DC converters and regulators to support the onboard devices, including the important communications functions (Bluetooth/Wi-Fi).
Typically, several voltage rails will be required internally within the wearable device, as the microcontroller, display and sensors are likely to have different voltage requirements. Many peripherals, such as the communication ICs and sensors, can spend most of their time in sleep mode and are only powered up when something is required of them. The microcontroller tends to work more of the time and, with modern devices, the current consumption can be budgeted to be around 40μA per MHz, showing that it can be a major user of power – and the clear relationship between performance and power used.
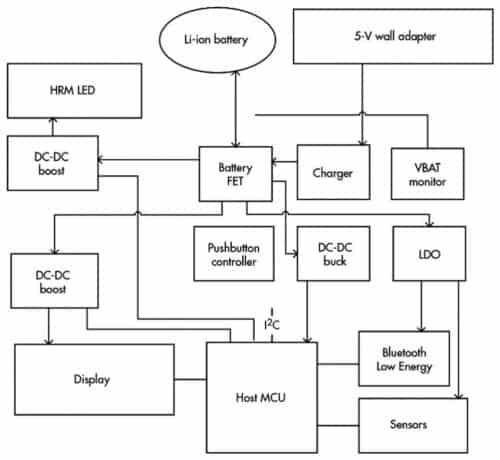
There are two primary methods by which PMICs generally perform DC-DC conversion. One method is via the onboard linear regulators which are often fully integrated into the PMIC chip and are able to be scaled for voltage levels. The second method uses a switching regulator and inductor combination – these are very efficient and are also scalable, but are most often discrete devices – not integrated.
Each different type of regulator has different characteristics, particularly with regard to efficiency, size and features/flexibility. In some cases, using regulators with very low quiescent current may be the best choice as they offer a very efficient solution for “always on” peripherals and devices. Efficient conversion should not be underestimated, as this can significantly reduce the active power when it is highest – especially when measurements are being made, or data is being received or transmitted.
Selecting the voltage regulator is a key decision for designers of wearable devices, due to the impact it will have on the efficiency. Designers must consider the power consumed while the device is active as well as when it is in standby mode, as both will impact battery drain.
One suitable LDO controller is the state-of-the-art ISL9016 from Renesas that provides up to 150mA per channel. This device exhibits a quiescent current of around 60μA and includes an electrostatic resistance (ESR) of up to 200mΩ.
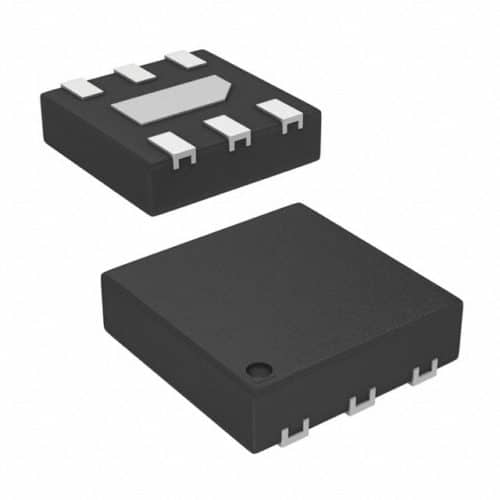
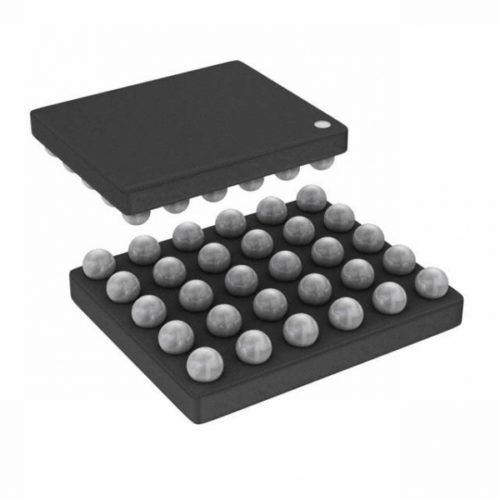
In general, switching regulators will always offer higher levels of efficiency than LDOs, although they do require several magnetic components (inductors) to create multiple voltage rails. As these passive devices can be bulky (and in many cases, costly) they are not desirable in wearable devices. An alternative to individual switching regulators that avoids the problems associated with increasing the component count and bill of materials is the single-inductor, multiple-output (SIMO) regulator.
Maxim Integrated’s MAX77650 SIMO buck-boost regulator IC can regulate as many as three output voltages over a wide range, thereby reducing the number of external components required, saving board space, weight and cost.
Batteries and Product Size
Managing battery drain and lengthening battery life is one of the primary challenges of wearable device designers. Small devices such as smart watches or fitness bands can often only accommodate a single-cell Li-ion battery with a voltage of 3.8V and a capacity of between 130mAh and 410mAh. The battery management and recharging system must closely monitor the current, voltage and temperature at all times while the device is in operation, and also while recharging. An ideal power management solution will ensure that the system consumes as little power as possible and recharges as rapidly as possible, while not exceeding any thermal limits.
One device designed for charging single-cell Li-Ion batteries as well as other chemistries, including lithium polymers, is Texas Instruments’ highly integrated BQ25100 PMIC. The device also allows the use of low-cost network adapters with a non-stabilized output.
Li-ion batteries are definitely the preferred technology for small rechargeable applications, and they have a larger market share than any other technology. However, the technology that beats them in terms of power, size and number of cycles is supercapacitors. These nanotechnology-based devices are slowly replacing rechargeable batteries for energy storage in wearable devices as available space continues to reduce in pursuit of ultra-sleek designs.
Supercapacitors offer several advantages over batteries, including the ability to work well with energy harvesting, to recharge within seconds and to offer an almost unlimited number of charge cycles. Murata’s ultra-thin DMH supercapacitors offer capacities of 35mF, a nominal voltage of 4.5V and an ESR of 300mΩ – all contained within a 20mm x 20mm x 0.4mm package.
Many companies are looking into the possibilities of using energy harvesting as a permanent additional energy source for wearable devices, removing some of the need for ultra-low-power designs. One area of study is triboelectric charging, whereby electric currents are created by two different layers of material moving against each other. The friction from the movement builds up an electrical charge on the materials. Placing the two materials between two conductors allows microwatts of power generation as the device wearer goes about their daily routines, adding highly useful energy to the onboard energy store.
Summary
Efficient design and architectures are crucial to modern wearable devices where the battery capacity is limited by the need for small size and low weight. Modern PMICs are valuable in segmenting the systems and providing the necessary voltage rails efficiently, with minimal external components. Looking to the future, supercapacitors will have a role to play, and looking even farther forward, energy harvesting techniques such as triboelectric charging may provide a useful auxiliary energy source.