Ultrasonic imaging is simple and cost-effective, especially for use in remote places, but it is not as good as CT or MRI imaging. However, with each passing day, and with technology getting better and better, software-based ultrasonic imaging systems are becoming better too. It is just a matter of time before these start replacing CT and MRI, according to the authors.
Ultrasound imaging has been around for many decades now. Regular enhancements in its features and capabilities, and the rapid miniaturisation of ultrasound devices has helped it thrive in the field of medical diagnosis. It started way back in 1794 when, Lazzaro Spallanzani, an Italian physiologist came to know about the phenomenon of echolocation (using ultrasound waves to hunt and trace path) among bats, which later formed the basis of ultrasonic imaging.
Further, in 1877, piezoelectricity was discovered by Pierre Currie and Jacques Currie. Piezoelectric effect is used in transducers for ultrasonic imaging; it converts electric current to longitudinal acoustic waves. In 1915, the first transducer was designed to detect objects under the sea. In early 1940s, first instance of using sonography for medical diagnosis came into light when a neurologist named Karl Dussik used it for detecting brain tumors.
In late 1940s came the first A-mode ultrasound device for detecting gallstones, and between 1949 and 1951, 2D B-mode ultrasound device was introduced. Later, in 1970s, further developments came in ultrasound devices in the form of Doppler ultrasound and Colour Doppler ultrasound. Introduction of more sophisticated ultrasound devices with 3D capabilities and M-mode visualisation took off in 1990s.
Ultrasonic imaging is done using acoustic waves of ultra-high frequency. The audible range of human ear is 20Hz-20 kHz. For ultrasonic imaging, acoustic frequencies of about 10MHz are used normally. There are two main reasons for choosing high frequencies for this operation:
- If normal audible frequencies are used for imaging, it may annoy the patients by creating loud noise, and that too in an environment where it is strictly undesirable.
- Higher frequencies suffer low losses due to various factors. So images captured using high frequencies will have better axial resolution.
Now, before going into the working, we must understand certain aspects of ultrasonic imaging. It is normally done in four modes, which are:
- A-mode. In this mode a single transducer is used to project, detect and plot the wave with respect to the depth of the target. Diagnosis of a specific tumor is also done using therapeutic A-mode ultrasonic imaging.
- B-mode. This is the type through which the echoed signal can be viewed as a 2D image on the screen. To achieve this a linear array of transducers is used to simultaneously scan through the targets by moving or swinging the transducer.
- M-mode. Known as Motion mode, in this, 2D images or B-mode scans of an organ are run in a sequence according to transducer position on a screen, so that information from multiple angles could be obtained to make more accurate conclusions.
- Doppler mode. This mode is generally used to visualise the body fluids that are in motion (usually blood). The Doppler Effect comes into picture as the moving body fluids change frequency of the echoes sent back. The amount of change in frequency indicates the speed of the fluid. As heart pumps blood in the body, it can be used to study the characteristics of it, and hence to detect any cardiovascular ailment.
Ultrasound components and working
The main component of an ultrasound device is a transducer, which converts an electrical signal to a longitudinal acoustic wave that travels through the body of the patient. It also catches the echoes reflected back by various organs.
The transducer is used in echo-scopic probes in a linear, sectorial or convex fashion to get different fields of view. For instance, an echo-scopic probe with linear array of transducers is used in situations where the window or area of access to the body is comparatively large.
The transducer is supplied with short-duration high-voltage pulses using a pulse generator. Compensating amplifiers are used to amplify the received weak echoes after reflection.
Digital processors and control units are used to process various signals that are transmitted and various signals that are received. A display unit shows the received signal.
The transducer sends out acoustic pulses, and each pulse’s transmitting time and receiving time is recorded by the transducer. As the received signals are the echoes that are reflected back from surfaces of the organs, or from different obstacles faced by the pulses, each echo varies in its intensity. This intensity of the echoes is displayed as a function of distance to reveal various shapes (organs).
As an acoustic pulse travels through different mediums it may get reflected, refracted, scattered, diffracted, absorbed or be interfered with. The reflection is necessary for ultrasonic imaging to work. As the pulse encounters different organs with different shapes and sizes, different densities, compressibility and different bulk modulus, these may weaken the echo, or may destroy the pulse completely.
The behaviour of pulses in various mediums has been studied, and it has been found that different targets reflect back different intensities of echoes. Based on this, different targets can be placed in three different categories:
- Hyper-echoic
- Hypo or anechoic
- Iso-echoic
The hyper-echoic targets reflect most of the pulse energy that falls at their surface. This means they reflect back almost all of the pulses that collide with them. The brighter regions in a 2D ultrasonic image can be characterised by these targets. Typical examples of such targets are bones, air and capsules.
The hypo or anechoic targets do not reflect pulses at all. They absorb all the pulses that fall on their surface. The darkest regions in the display signify these targets. Some examples of these regions are body fluids such as blood. These areas cannot be seen through ultrasonic imaging.
The third category, iso-echoic, is of the targets that reflect back some amount of echoes. These neither fully absorb nor fully reflect back the incident pulses. These areas can be identified as normal intensity (not too dark, not too bright) on the display. Examples include body fat and muscles.
As air can also attenuate acoustic pulses, a gel is applied on the patient’s body before ultrasonic imaging to minimise any trapped air between the probes and the body and maximise the output.
In Fig. 1, areas marked as 1 are hyper-echoic areas, those marked as 2 are hypo or anechoic areas, and areas marked as 3 are iso-echoic areas. Based on this information, we can presume that various targets on the display can be perceived through varying levels of intensities.
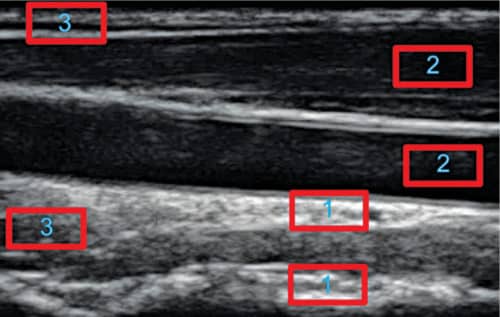
Although discrimination of an ultrasound equipment is very important, if two reflecting targets are very close to each other, the spatial resolution can play a very important role in recognising them as separate. In this regard, we can define two main types of spatial resolutions in ultrasound as (a) lateral resolution, and (b) axial resolution.
As mentioned earlier, the penetration of an acoustic beam depends on the frequency, density, compressibility and bulk modulus of the medium. At higher frequencies the diffraction and other losses decrease, but penetration also decreases. So, if density and compressibility of a medium is increased, it impedes the penetration of the wave. But if stiffness or bulk is increased, it facilitates better penetration of wave into that medium.
If two reflectors are parallel to each other and lie in direct scan line of ultrasonic wave, the minimum distance that can be recognised and differentiated is called the axial resolution or longitudinal resolution.
As attenuation,
where d is the depth of the target that reflects back, is the frequency (in MHz) and 0.5dBcm–1 is the assumed attenuation coefficient for travelling ultrasonic wave in soft tissue.
It can be seen that the attenuation is directly proportional to the depth of target reflector and frequency of the ultrasonic wave. This means that axial resolution is high when frequency of the acoustic wave is kept high and spatial pulse length (number of cycles in a pulse×(wavelength)) is kept short. The number of cycles depends on the damping nature of the transducer, which in turn depends on the geometry of it. So, in case of deep-target structures, low axial resolution is obtained.
When the target areas are perpendicular to the scanning ultrasonic wave, the distinguishing ability between two reflectors can be regarded as lateral resolution. This resolution decreases with the distance as the ultrasonic wave diffracts. It depends directly upon the near-zone length. Near-zone length is inversely proportional to wavelength of the acoustic wave. It also depends upon the frequency, width and damping factor of the transducer.
Applications
Ultrasounds can be used in many applications in the field of medical diagnosis, such as:
- Fetus size measurement to determine the due date of delivery
- To check number of fetuses, the position of placenta and the sex of the baby
- For checking inside the heart for any cardiovascular ailment
- To measure blood flow (Doppler mode) through heart and kidney
- To detect prostate cancer in kidney
- To detect stones in gall bladder or kidney
- To detect tumors in ovaries and breasts
- To detect the fatty liver by observing its size
It is also used together with other modalities to do some complex medical analysis of the patient.
Pros and cons of ultrasound imaging
Ultrasound imaging possesses some advantages over other imaging modalities like:
- First and foremost being that, it is a non-invasive method that does not require any cut in the patient’s body.
- It does not expose patient’s body to any harmful radiations like x-rays or CT scans, which is very important for pregnant mothers.
- It captures soft tissues very well, unlike x-rays.
- It is becoming less expensive and more accessible with rapid miniaturisation.
But ultrasound imaging has some disadvantages also. For instance, it has been found that pregnant mothers having frequent ultrasound examinations may develop risk of delivering underweight babies. And that the local temperature of tissues increases due to frequent collision with ultrasonic energy.
Besides, there are some challenges that still exist in this field. One such challenge, addressed in this article, is the low spatial resolution of ultrasound images. In addition, ultrasonic images are also prone to Gaussian and speckle noise. There are some artifacts, like mirror image artifacts, which also impact ultrasound imaging.
To address all these challenges, similar strategies can be adopted such that maximum number of problems can be solved at minimal cost.
Some recent trends
Ultrasonic imaging has evolved a lot in the past few decades. Performance enhancement and addition of new features have frequently been done in this field. Rapid commercialisation of hand-held ultrasonic imaging devices has made this modality even more popular. The smaller devices are easy to carry and so can be taken to remote rural places for point-of-care diagnosis.
Work has been going on to reduce the noise in the ultrasonic images. For that, Tissue Harmonic imaging has been introduced in which reconstruction of image is done using second harmonic of echo from the tissue.
Recently, 3D and 4D ultrasonic imaging has also been introduced to further enhance the experience of visualisation of motion of the heart wall. The 4D technology is aimed at competing with imaging modalities like CT and MRI in terms of resolution and image clarity. Incorporating ultrasonic equipment with GPS has enabled needle positioning feedback in real time in case of interventional procedures.
In addition, nowadays, ultrasonic images of an area are fused with the images of other modalities, like CT and MRI images of the same areas, to further enhance the diagnosis experience.
Image super-resolution
On first observation of the ultrasonic image in Fig. 2, it can be seen that ultrasonic images suffer low spatial resolution due to weak received echo signal from the target reflectors, along with a combination of Gaussian and Speckle noise. These drawbacks can be hard to address through any hardware changes.
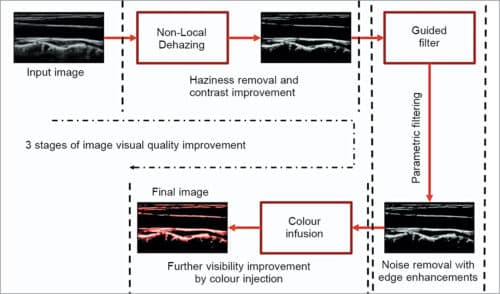
Apart from these demerits, ultrasonic images have very low resolution details as compared to CT and MRI. When any hardware changes are attempted, the equipment can become expensive and may pose some other unforeseen problems. An inexpensive and effective solution is the correction of images through software; if it poses any problem the system can be easily modified as per requirement.
In this article, we propose a super-resolution method to enhance the quality and hence visual perceptiveness of the ultrasonic images by developing a hybrid approach. Flow chart for the proposed method is shown in Fig. 2.
We start with a low-spatial-resolution ultrasonic image and apply non-local dehazing to it as the input image appears to be a little bit hazy and blurry. Due to this, there is very less visibility and contrast; also there is lack of proper luminance.
The non-local dehazing method is a linear, non-trained method that efficiently recovers the true characteristic image from the hazy one. It also handles the noise with care and makes the contrast better.
Fig. 2 shows the result after non-local dehazing. The resulting image has good contrast and better luminance. The details are revealed further when we remove the noise using the Guided filter. Guided filter is an edge preserving smoothing filter that effectively removes the noise while preserving the edges with high contrast details. It is an explicit image filter that uses a reference image known as the guidance image to look for the edges to be preserved.
In the proposed method, a square window of 16 is chosen to make operation fast and the edge selectivity controlling parameter of 0.0016 is chosen. The edge selectivity parameter is chosen to be low because for large values of the parameter the filter converges to a normal box filter with lost edge preserving properties. The guided filter brings out subtle details and makes them more visible as seen in Fig. 2.
It is well known that colour images are more visually appealing than grayscale images. The additional colour component in the images helps the human perceptual system to perceive better. So a red blood colour is injected into the filtered image to make it more visually appealing. The final image looks very informative and gives high and accurate spatial as well as temporal resolution.
This method is applied on three different datasets and objective evaluation of the method is done on the basis of peak signal-to-noise ratio (PSNR), which calculates overall signal strength over the noise strength and mean square error (MSE) that calculates the distance of corresponding pixels between final image and reference image (input image). The visual results of the proposed method on three different datasets are shown in Figs 3, 4 and 5. The objective evaluation results are shown in Figs 6 and 7.



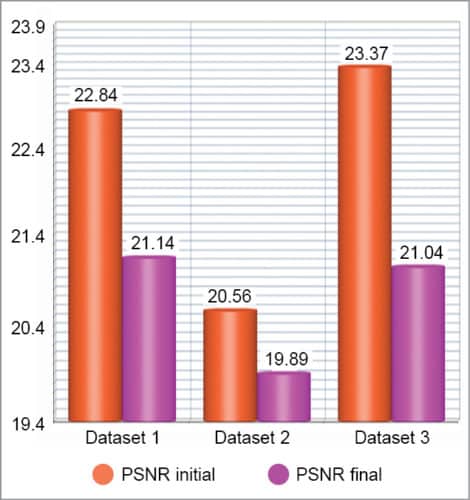
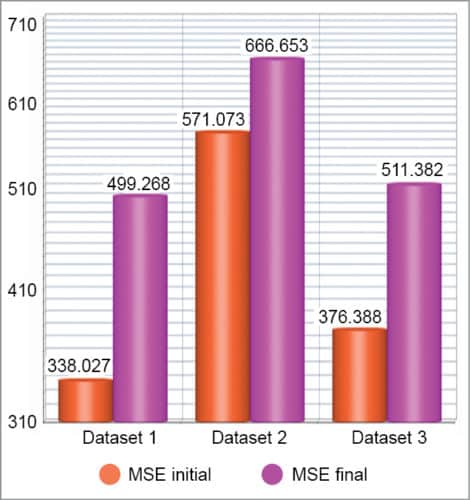
Although the objective evaluation results show decrease in PSNR and increase in MSE, the visual results have improved a lot, which is evident from the final results obtained. The purpose of providing super-resolution to ultrasonic images is achieved through this proposed architecture, which can be applied seamlessly to any ultrasonic image without adding any extra cost of implementation.
It is clearly visible that the proposed architecture has a strong prospect of extending the efficacy of ultrasound images with high spatial and temporal resolutions. The method clearly retrieves the hidden details of the common carotid artery.
Conclusion and future
It is well known that CT and MRI imaging have a very distinctive place in the field of medical imaging and diagnosis. These images have much better resolution and contrast, and provide comparatively more information content about the target areas than ultrasonic images, but these are also much costlier than ultrasonic imaging.
A simple and cost-effective solution to this is ultrasonic imaging. It does not provide as good image as a CT or MRI, but with each passing day and with technology getting better and better, ultrasonic imaging systems are also becoming better.
It may not be wrong to think that, focusing primarily on ultrasonic imaging and making it better through various means will help medical fraternity to reach more widespread areas where bringing other imaging technologies is difficult. This article is one such effort to improve ultrasonic imaging at the software end, so that imaging quality can be improved at a fraction of cost.
Ayush Dogra and Sanjeev Virdi belong to Biomedical Instrumentation Unit, CSIR-CSIO, Chandigarh.
Apoorav Maulik Sharma is from Department of ECE, UIET, Panjab University, Chandigarh, and Bhawna Goyal is from Department of ECE, Chandigarh University, Punjab