Demand for medical devices is growing at a fast rate as people become more and more health conscious with increasing government-aided social healthcare schemes and an increasing number of patients moving from hospitals to their homes for nursing and rehabilitation.
An autonomous, non-invasive medical device that performs a specific medical function such as monitoring or providing support over a prolonged period of time is called a wearable medical device. The term wearable implies that the support environment is either the human body or a piece of clothing. Handheld and portable devices are, strictly speaking, not wearable devices. However, in a broader sense, such medical items as splints, bandages, eyeglasses and contact lenses are commonly considered as wearable medical devices.
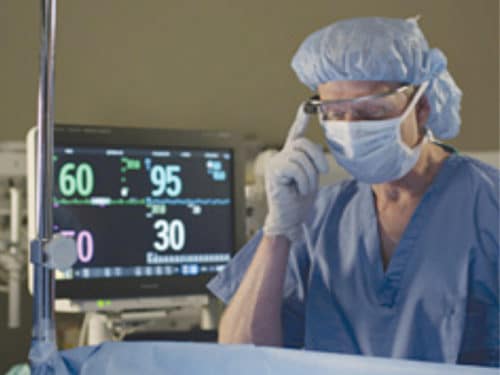
Usually, the term wearable medical device simply stands for a sophisticated device with the capability for integrating mechanical functions with microelectronics, computing capabilities and, in some cases, a degree of artificial intelligence (AI). Such a device has the capability to collect, store, process and analyse data to provide necessary feedback, and instantly send alerts or medical decisions to patients and medical supervisors.
An example of a modern wearable medical device is sleep apnea monitor used for infants susceptible to sudden infant death syndrome. The device consists of a respiratory effort belt worn by the sleeping infant, which provides an alert when breathing patterns become a cause for concern.
Another example is of a navigation device, which is based on global positioning system (GPS), and is used to assist the visually-impaired in navigation, way-finding and obstacle-avoidance.
Wearable devices are designed for specific purposes, such as monitoring vital medical parameters, providing long-term support, medical aid and rehabilitation assistance to patients with temporary or permanent disabilities, non-invasive sensing, communicating with patients and also for receiving feedback from them. Medical parameters being monitored include electrocardiography (ECG), respiration, skin temperature, pulse, blood pressure or blood oxygen saturation, body kinematics, as well as sensorial, emotional and cognitive reactivity for providing effective treatment of cardiac diseases, asthma and diabetes.
Background
The focus in personal healthcare has shifted from hospital care to home care and further to healthcare on-the-move. As a result, usage and popularity are rapidly shifting from fixed medical devices and systems to portable ones and ultimately to wearable devices.
First-generation devices needed user involvement to obtain and record data, which was processed offline; for example, a heart holter, which is used for monitoring and recording cardiac activity. Electrodes placed on the patient’s chest are connected to a small battery-operated recording monitor worn by the patient. The patient records the activities undertaken during the period in a diary. This data is later analysed on a computer to correlate irregular cardiac activities, if any.
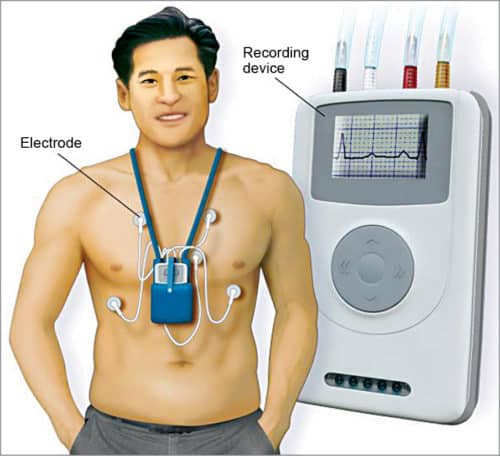
The need for obtaining real-time information and alerts led to the development of the next generation of wearable medical devices. These devices provide real-time wireless telemetry, feedback, medical alerts and clinical decisions by collecting, storing, processing and analysing clinical data. These have the ability to display and transmit information relating to health condition using non-invasive electronic, mechanical or biochemical sensors for monitoring physiological and kinesiological parameters. Advancements in micro sensor technology, computing technology, wireless communication capabilities and miniaturisation are assisting in the innovation of advanced wearable medical devices.
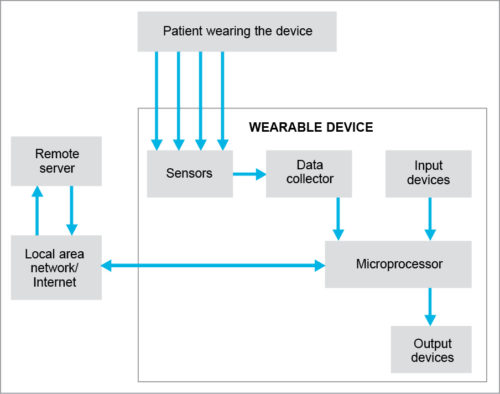
Development of modern-day wearable devices was initially taken up for the US space programme when NASA needed to continuously monitor the essential medical parameters and physiological responses of astronauts in space. Further development was made by the US Army for developing wearable devices for use in battlefield with operational capabilities such as monitoring the soldier’s health condition and his/her ability to fight.
A medical status monitor was used to read blood pressure, pulse, respiration and blood oxygen level, and transmit alarms along with the soldier’s GPS location and severity of the injury. Later, improvements resulted in decision-making capability along with monitoring support.
Developments and improvements in the field are constantly taking place to provide greater sophistication and facilities by using emerging technologies such as AI and nanotechnology. Wearable medical devices are now freely available commercially for high-risk patients, athletes and for research purposes.
Main constituents of wearable medical devices
A wearable medical device receives inputs from sensors and the data collector. The microprocessor, which is the heart of the wearable medical device, provides data processing, data storage and wireless communication with the remote server. Sensors positioned on the body of the patient monitor him/her and the surrounding environment parameters, and feed this data to the data collector.
The patient enters the information into the microprocessor using input devices, such as keypad, touchscreen, etc. Collected data is fed to the microprocessor. Remote server is the link between the wearable device and the outer world. These communicate with each other through local area network, Internet or telemedicine facilities. Output devices provide display/feedback/alert or implement required actions, such as functional stimulation and drug delivery. Components used are miniature, light, durable, comfortable, and provide the necessary functional reliability and safety at low cost.
Technologies involved
Technologies involved are discussed next.
Computer and communication technologies
Computer and communication technologies provide data acquisition, storage and processing, and also communication with the external world. Advanced devices use user-programmable integrated circuits, field programmable gate arrays and complex logic devices. Integration of hardware and software ensures the required processing speed.
Data storage capacity in the range of 8MB to 3GB is normally used to retain data indefinitely without battery. Input/output technologies facilitate human-computer interaction without hindering normal body activities. Technologies enable the use of such input-output devices as miniaturised microphones and speakers, voice-recognition software, body-mounted keyboards, miniaturised pointing devices, touchscreens, handheld keyboards, miniature liquid crystal or LED displays, clip-on monitors and embedded monitors in glass frames.
Medical sensors
Sensors provide digital electrical output that can be easily processed. These have to be wearable, non-invasive, capable of offering painless and comfortable measurement, and prevent infections and contamination. Attached to the human body, these monitor physiological or kinesiological conditions that include heart and muscle activities, respiration, skin temperature, blood pressure and body posture, movement and acceleration.
Selection of sensors is based on specific functional requirements and operating conditions. There is frequently a trade-off between size, cost and power consumption, and durability and reliability of devices in the intended operating environment.
Peripheral sensors are used to monitor information from the outer environment and include miniaturised cameras, microphones, contact sensors, environmental sensors, and location- and geographic-information-monitoring sensors.
Non-invasive sensors like electrodes are used for detecting skin surface potential in bioelectric monitoring such as ECG, electroencephalography (EEG) and electromyography (EMG). Thermistor sensors are used for monitoring skin surface temperature; piezoelectric sensors are used for monitoring heart rate and respiratory effort.
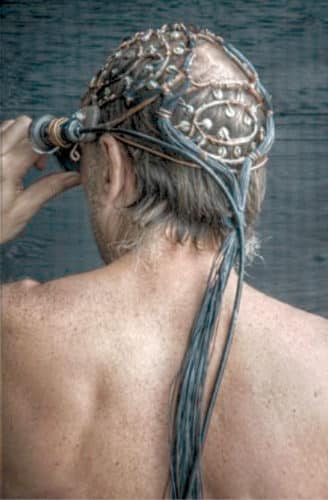
Photoplethysmography (PPG) sensors are used for detecting blood volume changes of skin area. Infrared light emitted into skin is absorbed proportional to blood volume and backscattered corresponding to the variation of blood volume. Scattered light is measured to compute blood volume variation. Galvanic skin sensors are used for detecting skin conductance.
Oximetry sensors are used for detecting blood oxygen saturation and heart rate. An infrared emitter/receiver worn on the finger or ear lobe transmits light through skin. Continuously varying concentrations of oxygen in blood are measured by the receiver, and heart rate is determined from the variation in concentration over time.
Several other sensors are available such as micro-electromechanical sensors (MEMS) and microelectronic biosensors (MEBS). MEMS are piezo-resistive silicon pressure sensors based on Wheatstone Bridge, and are used to measure blood pressure, respiration and acceleration.
MEBS are of two types: calorimetric or electrochemical biosensors. Calorimetric biosensors are used for monitoring enzymatic reactions by detecting heat of the biological reactions. Electrochemical biosensors are used to monitor enzymes such as glucose, lactate and urea.
Potentiometric sensors are used to measure electrical potential between two electrodes at zero current flow. Amperometric biosensors are used to measure current at an electrode. Research efforts are on to produce multi-biosensor elements, integrating amperometric and potentiometric sensors on one substrate to form an integrated laboratory-on-a-chip and to develop wireless biomedical sensors.
e-clothing and accessories
e-clothing uses combined textile and electronic technologies. In e-clothing, electronic sensors, circuits or piezoelectric materials are attached, included or integrated into conventional clothing or accessories to store, process, retrieve and send information. Examples are conductive fabrics woven with silk fibres that have fine gold, silver or copper wrapping. A fabric keyboard that can be crushed or washed, and smart garments with integrated processors and transmitters are other examples.
Fabrics have also been developed using a micro-supercapacitor with conductive threads to store charge in self-powered smart garments. Special sewing technology creates a flexible mesh of aligned electrodes on a textile backing for a solid-state wearable device with ability to store charge that can monitor health in real time.
Examples of accessories are smart shoes that monitor the impact on ground to detect the runner’s pace and send information through the Internet to the coach; accessories such as belts, jewellery, gloves and wrist watches with sensing and computing components; head-mounted eyeglasses-based displays; and multimedia systems with wearable cameras, microphones and earphones.
Design challenges
Three significant features differentiate wearable medical devices from other medical devices. First is their wearable nature, which requires that these should be usable in diverse environmental conditions, be comfortable, not noticeable, autonomous, lightweight and compact.
Second is that the patient must be actively involved in their setting up, operation and usage.
Third is that these need to be commercially-viable and economically-affordable for wide acceptance and usage.
These features pose a number of design challenges, as explained below.
Physical challenges
The weight, shape and size play a significant role in making these suitable for their purpose, use and acceptability from the point of view of comfort and fashion. Their relationship and interaction with the human anatomy and the work environment are also important. The following needs to be considered while designing a wearable device:
- The shape, material, texture and colour of the wearable device meets culture and context of use, and the device is comfortable and acceptable to users, easy to set up and operate.
- Weight of the device is distributed evenly on the body, avoiding any effect on natural body movements and balance.
- An appropriate location on the human body to provide a comfortable and steady fit, freedom for movement of joints, and protection from injury and irritants.
- Sensors are non-invasive, avoiding direct skin contact, providing a secure attachment to body without causing any skin irritation, and not getting influenced by physiological responses such as vibrations, sweating and prolonged use.
- Devices do not heat up body parts or trap heat.
- Long-term effect of usage is minimum and acceptable.
Technical challenges
These include:
- Data has to be acquired by the device as direct input from the user using keys, touchscreen or speech recognition software, or as output of sensors or through wireless data transfer.
- Analogue inputs have to be converted into digital data before transfer within the device.
- Alterations in contact resistance between the electrodes and skin over time do not affect input data. Environmental factors, noise, ambient temperature and humidity do not influence reliability of acquired data.
- Features such as noise filtering and signal distortion correction, data compression and signal processing for real-time response.
- The design needs to optimise data transfer rates, transmission range, interference between different telecommunication protocols, compatibility problems between devices and power consumption.
- Total power requirement and size of power sources considerably affect total size and weight of the device. There is always a trade-off between device performance and battery life for the intended period of use. Advancements are being made for utilising biologically generated energy, or energy generated by body movements.
Other challenges
Besides physical and technical, some other challenges are:
- The method used for providing alerts and feedback—visual, auditory or tactile—be relevant, comprehensible and reliable. As the stored medical information is personal and private, it should be accessible only to authorised users. Encryption techniques and levels are appropriate, reliable and balanced with the power capacity of the device.
- The device be user-friendly, especially when in use by inexperienced or uneducated users, to ensure safety of the patient during usage without causing any harm to the patient, or creating nuisance and inconvenience (such as skin rashes).
- Reliability of outputs and results is an important factor. Non-controlled environment such as varying humidity and ambient temperature, and physical factors such as sweating, vibration, or body movements should not affect reliability.
- Devices should comply with applicable standards, codes and regulations to ensure their acceptability, safety and reliability. International standards are available such as ISO 11073 and IEEE Standard 1073.
- These must meet legal, regulatory and ethical requirements.
- Costs including professional fee, cost of emergency handling, training to be imparted to the patient to setup and use, and the maintenance, should be within the easy reach of patient and commercially viable.
Applications
A range of wearable medical devices are readily available for use by patients and healthcare providers. These can be divided broadly into two categories: medical monitoring devices and medical rehabilitation devices.
Wearable medical monitoring devices
These devices are designed for non-invasive sensing, monitoring vital medical parameters, communicating with patients, and providing feedback and instant alerts, or medical decisions to patients and medical supervisors. Advanced devices monitor athletes during exercise and performance, and patients within the healthcare environment. A few examples are:
Wearable armband
A wireless body monitor worn on the arm for collecting physiological and lifestyle data such as movements made, heat flow, skin temperature, heart rate, sleep logs, etc.
Vivometrics LifeShirt
A non-invasive ambulatory monitoring garment with embedded sensors, which is used to monitor up to forty such physiological parameters as heart rate, breathing and lung capacity.
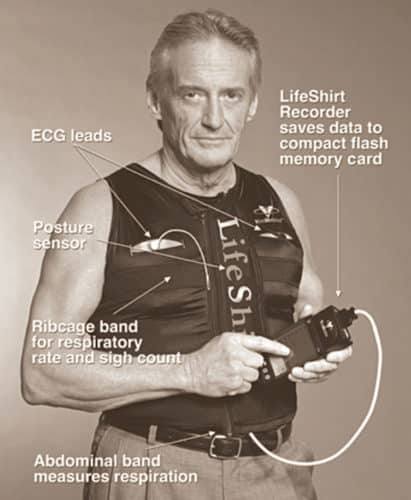
Polysomnograph (PSG)
Also called sleep tracker, PSG is a wearable multi-channel data recorder body patch worn on the chest of a patient to record ECG, EMG, electrooculography, EEG, chest effort, pulse oxymetry, body position, airflow, etc.
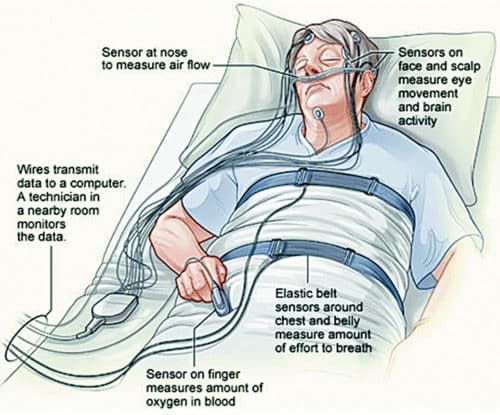
Wearable glucose monitor
A wrist-worn, non-invasive blood glucose monitoring device used by diabetic patients to provide readings every fifteen minutes by extracting glucose through skin via reverse iontophoresis.
Stress monitoring device
A wearable device used by drivers for determining stress levels while driving by monitoring/measuring parameters such as skin conductance, respiration, muscle and heart activity, and capturing facial expressions.
Wearable device for athletes
A device used for real-time monitoring of athletes’ movements, changes in position of their body, respiratory effort, heart condition, temperature, blood pressure and detail of any injury sustained. Real-time feedback is available to athletes and coaches via a virtual reality (VR) interface, which enables them to improve performance.
Wearable medical rehabilitation devices
These devices provide long-term support, medical aid and rehabilitation assistance to patients with temporary or permanent disabilities. Some representative examples are:
Rehabilitation device for nerve stimulation
It is used for therapeutic purposes to block pain by providing functional stimulation through electrical impulses to the patient.
Wearable motion analysis device
The device is used as a diagnostic tool as well as a biofeedback device during rehabilitation therapy to monitor patients with balance disorders.
It is also useful as a fall-prevention aid for fall-prone elderly persons. Triaxial digital accelerometers are attached on an eyeglass frame for measuring head motion and to a belt at the waist to monitor body balance disorders. A handheld control provides real-time visual and/or auditory feedback to the user and communicates patient status to a remote clinician.
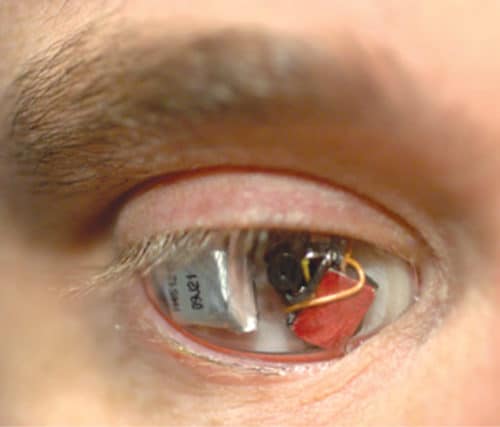
Wearable device for healing fractures
The device is used for accelerating the healing process of long bones for patients with open fractures. A pair of miniature ultrasound transducers is implanted into the affected region and is wirelessly connected to a unit located in a clinical environment. The device initiates low-intensity pulsed ultrasound therapy sessions, carries out ultrasound measurements and communicates the data to a centralised unit.
The future
Basic wearable medical devices such as headphones, hearing aids, health-bands help improve the quality of life. Functional aids used by mentally- or physically-disabled and chronically-ill persons provide higher levels of independence, and enable their social and vocational participation.
Technological advancements have made available wider data memory capability, augmented reality (AR)-based head-mounted displays, AI-based decision-making capability and improved communication. Advanced and superior components and sensors are helping in improving functionality, wearability, reliability and security of devices.
Devices are being integrated with implantable devices to provide real-time drug delivery, functional stimulation of the nervous system and heart management. Futuristic wearable devices will include automatic infusion of insulin when blood sugar levels are high, serve as implanted micropump assisted drug delivery systems, DNA diagnostics implants, artificial retinas, and disposable sensors for monitoring glucose, urea, sodium, and calcium.
An advanced GPS-based wearable navigation and guidance system for visually-impaired patients is under development to assist them in moving to the desired destination. The system includes a GPS receiver to locate the patient in a spatial GIS database, a compass for determining orientation, a cellphone for communicating with a central server, and a speech synthesiser to make available to the user information about nearby streets and other objects of interest.
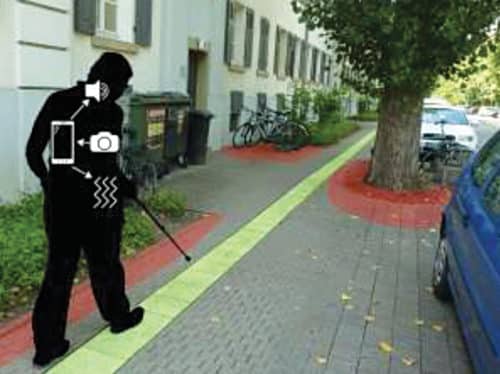
Stringent requirements for clinical testing, applicable legislation and regulations, and potential threats of litigation are some of the limitations responsible for the long period taken from conceptualisation to commercial availability of wearable devices. Wearable medical devices are treated more as complementary rather than an alternative to main hospital equipment. However, their ever-increasing capability combined with their practical benefits in providing unconfined medical monitoring, assistance in rehabilitation, improvement in the living conditions of chronically-ill patients and point-of-care service in remote locations make them highly useful.
These devices have a distinct advantage in providing telemedicine services, data transfer security and easy integration. These are making available vast useful information and data for research purposes, and providing effective health services in emergency situations.
Demand for medical devices is growing at a fast rate as people become more and more health conscious with increasing government-aided social healthcare schemes and an increasing number of patients moving from hospitals to their homes for nursing and rehabilitation.
Deepak Halan is associate professor at School of Management Sciences, Apeejay Stya University.