In the last few years, performance of organic solar cells has significantly improved. Further improvements are needed for the further development of organic photovoltaic cells. This article reviews the basic working principle, commercialisation and the fate of organic solar cells. Importance of high power conversion efficiency models for organic solar cells and the future development in the field of nanotechnology are also discussed
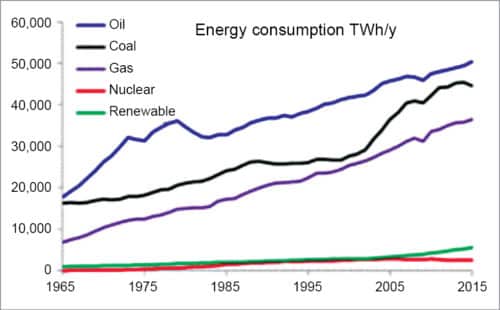
Today, we are all aware of the fact that fossil fuels such as natural gas, coal and oil are finite resources available for a finite time. Excessive worldwide usage of these resources has introduced conflicts and such modern concepts as global warming with the consequence of the depleting ozone layer. And total demand for energy is increasing day by day.
An organic solar cell is a type of photovoltaic that uses organic electronics—a branch that deals with conductive organic polymers or small organic molecules for light absorption and charge transport to produce electricity from sunlight using photovoltaic effect. Organic solar cells are cheap, resulting in low production costs to form large volumes. Due to the high optical absorption coefficient () of organic molecules, large amounts of sunlight can be absorbed easily and efficiently.
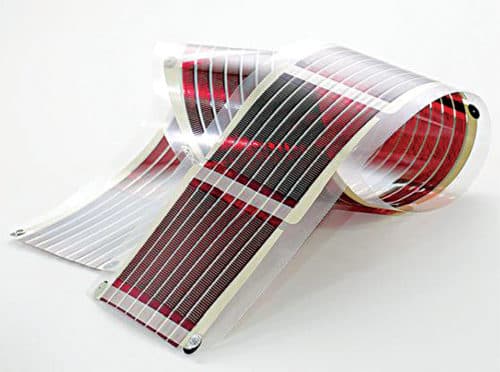
Compared to conventional silicon solar cells, organic solar cells are lighter in weight, easily disposable, convenient to fabricate, potentially transparent, flexible and come with a lower manufacturing cost.
After considering the above merits, organic solar cells find wider applications than conventional ones. Organic photovoltaics can be installed on windows or rooftops, as shown in Fig. 3.
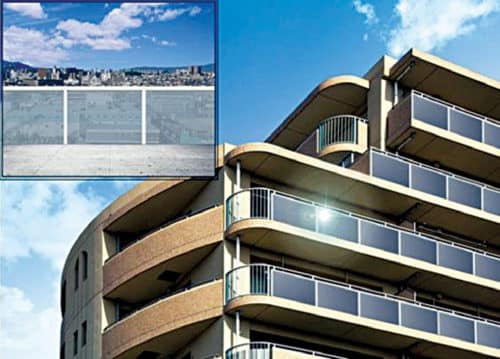
Fig. 4 shows transparent solar organic solar cells designed and manufactured by a German company called BASF. The firm produces creative organic solar organic photovoltaics and has published several researches in the field.
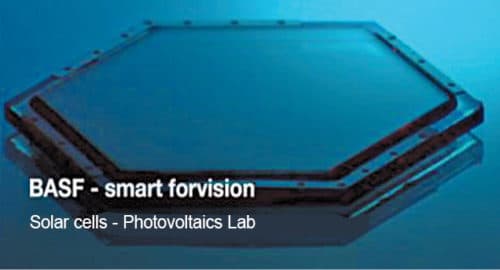
BASF has successfully manufactured transparent organic bulk hetero junction solar cells, and is now trying to expand this activity.
The physics of organic photovoltaics
Actual structure of an organic photovoltaic is made of two materials: fullerene (nanomaterial carbon) and P3HT polythiophene, which is basically a polymer.
A common characteristic of small molecules (fullerene) and polymers (P3HT) (Fig. 5), used as the light-absorbing material in photovoltaic, is that both have a large conjugated system. A conjugated system is formed where carbon atoms covalently bond with alternating single and double bonds. These hydrocarbons’ electrons pz orbital delocalise and form a delocalised bonding π orbital with a π* ant bonding orbital. The delocalised orbital is the highest occupied molecular orbital (HOMO), and π* orbital is the lowest unoccupied molecular orbital (LUMO).
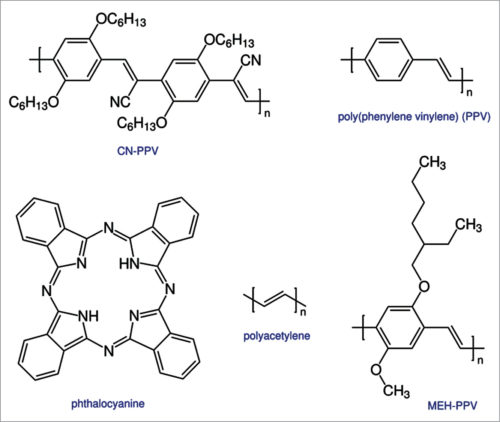
In organic semiconductor physics, HOMO takes the role of the valence band, while LUMO serves as the conduction band. Energy separation between HOMO and LUMO energy levels is considered as the band gap of organic electronic materials, and is typically in the range of leV to 2.3eV.
All light with energy greater than the band gap of the material can be absorbed, though there is a trade-off for reducing the band gap, as photons absorbed with energies higher than the band gap will thermally give off their excess energy, resulting in lower voltages and power conversion efficiencies. When these materials absorb a photon, an excited state is created and confined to a molecule or a region of a polymer chain.
Excited state can be regarded as an exciton, or an electron-hole pair bound together by electrostatic interactions. In photovoltaic cells, excitons are broken up into free electron-hole pairs by effective fields. Effective fields are set up by creating a heterojunction between two dissimilar materials. In organic photovoltaic, effective fields break up excitons by causing the electron to fall from the conduction band of the absorber to the conduction band of the acceptor molecule. It is necessary that the acceptor material has a conduction band edge that is lower than that of the absorber material.
Bulk heterojunction solar cell
Bulk heterojunction has an absorption layer consisting of a nano-scale blend of donor and acceptor materials. Bulk heterojunction is most commonly created by forming a solution containing the two components, casting (that is, drop casting and spin coating) and then allowing the two phases to separate, usually with the assistance of an annealing step.
The two components self-assemble into an interpenetrating network connecting the two electrodes. These are normally composed of a conjugated molecule-based donor and fullerene-based acceptor. The non-structural morphology of bulk heterojunction tends to be difficult to control but is critical to photovoltaic performance.
After the capture of a photon, electrons move to acceptor domains and are then carried through the device and collected by one electrode. Holes move in the opposite direction and are collected on the other side. If dispersion of the two materials is too fine, it will result in poor charge transfer through the layer.
Most bulk heterojunction cells use two components, although three-component cells have been explored. The third component, a secondary p-type donor polymer, acts to absorb light in a different region of the solar spectrum. This in theory increases the amount of absorbed light. These ternary cells operate through one of three distinct mechanisms: charge transfer, energy transfer or parallel linkage.
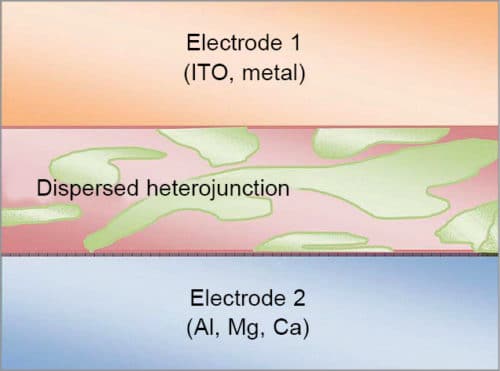
In charge transfer, both donors contribute directly to the generation of free charge carriers. Holes pass through only one donor domain before collection at the anode.
In energy transfer, only one donor contributes to the production of holes. The second donor acts solely to absorb light, transferring extra energy to the first donor material.
In parallel linkage, both donors produce excitons independently, which then migrate to their respective donor/acceptor interfaces and dissociate.
What is inside organic solar cells is called bulk heterojunction solar cells. While making organic solar cells, polymer is taken in its semi-solid form and carbon is then mixed in it, so it becomes a semi-solid mixture such as ink of a fountain pen. This ink is made to settle for a considerable amount of time. As the ink settles, it looks exactly like what is shown in Fig. 7. This is how bulk heterojunction is made practically.
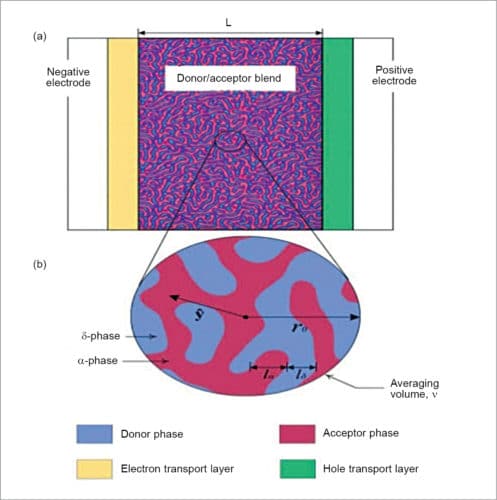
Power conversion efficiency (η)
One of the major issues surrounding polymer solar cells is the low power conversion efficiency (PCE) of fabricated cells. To be considered commercially-viable, photovoltaic solar cells must be able to achieve at least ten to fifteen per cent efficiency, which is already much lower than of inorganic photovoltaics. However, due to the low cost of polymer solar cells, ten to fifteen per cent efficiency is commercially-viable.
PCE (η) is proportional to the product of short-circuit current (ISC), open circuit voltage (VOC) and fill factor (FF).
η=(VOC)×(ISC)×Fill factor
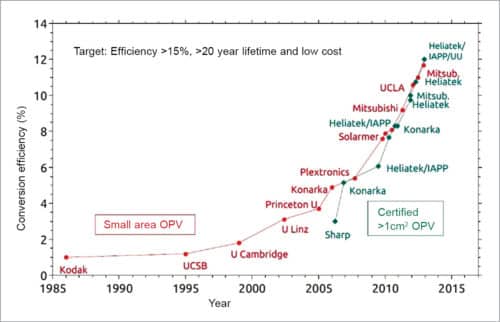
Recent advances in polymer solar cell performance have resulted from compressing the band gap to enhance short-circuit current while lowering HOMO to increase open-circuit voltage. However, photovoltaic solar cells still suffer from low fill factors (typically below 70 per cent). But, as of 2013, researchers have been able to fabricate photovoltaic solar cells with fill factors of over 75 per cent. Scientists have been able to accomplish this via an inverted BHJ and by using non-conventional donor/acceptor combinations.
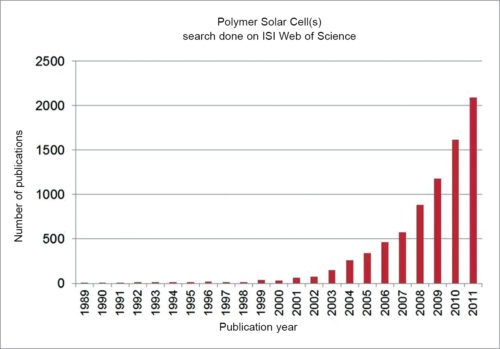
Commercialisation
Many multinational companies are interested in this newly emerging solar technology. Researchers are putting in a lot of effort in this field to make it better and efficient. Today, efficiency of an organic solar cell is ten per cent, which is way behind silicon solar cells’, but it is in progress. A number of organic photovoltaic manufacturing companies have been established and are receiving good feedback from the market. Some of them have launched their products commercially in the market, too.
Advantages
Some advantages are given below:
- Low weight and flexibility of photovoltaic modules
- Semi-transparency
- Easy integration into other products
- New market opportunities, for example, wearable photovoltaics
- Significantly lower manufacturing costs compared to conventional inorganic technologies
- Manufacturing of organic photovoltaics is a continuous process using state-of-the-art printing tools
- Short energy payback times and low environmental impact during manufacturing and operations
Most of the advantages listed above also apply to solar cells based on vapour-deposited small-molecule absorbers. This suggests that organic photovoltaics have the potential to be a disruptive technology within the photovoltaic market. The bright outlook has initiated many research and development activities, and substantial progress has been made in increasing the PCE of solutions-processed organic photovoltaic in the last few years.
Conclusion
To summarise, bulk heterojunction organic solar cells represent a promising technology that could be an important player in the future photovoltaics market. Substantial research and development efforts are required to bring the technology to better performance levels.
The flexibility offered by organic chemistry to design semiconductors and engineer interfaces to other inorganic or organic materials would offer various opportunities to explore third-generation concepts. This advancement in technology will hopefully prove fruitful for a green earth and, subsequently, reduce greenhouse gases.
Inventions and researches in organic solar cells are noteworthy due to which the predicted future of organic solar cells is extremely bright. Many multinationals are interested in investing in such constructive ideas. Tesla is trying to make rooftops using solar cells, which will be a good source of energy and rigid enough to protect roofs as well.
Also, as mentioned above, organic solar cells are at present semi-transparent, and efforts are being made to make these entirely transparent for installation in home windows. Organic solar cells will then play a vital role in constructing a better and eco-friendly future.
Shreyas Sonavani is pursuing diploma in electrical and electronics engineering from Department of Electrical Engineering, MET’s Institute of Technology (polytechnic)
To read other interesting articles related to solar technology: click here