Present-day commercial silicon solar cells have about 22 per cent efficiency. One of the most efficient ways to increase photovoltaic performance is integrating heliotropism, that is, the ability to track the Sun across the sky, using solar cells for more efficient photon collection.
The ability to follow the source of sunlight is called heliotropism (helio relates to the Sun and tropic to loving), whereas phototropism is the ability to track light. Sunlight causes the destruction of a plant hormone group called auxins that build up in the cells on the shady side. Auxins, like indole acetic acid (IAA), promote cell growth and division. Hence, there is much more growth of longer cells and a higher number of cells on the shady side.
Uneven growth pattern makes one side longer than the other and forces the stalk to turn. As shown in Fig. 1, in the first and second sections, when sunlight is overhead, auxins (IAA) molecules are evenly distributed in the stalk of the sunflower. Once sunlight shines at an angle, auxins move to the far side where there is shade, and induce elongation of cells on that side, as shown in the third and fourth sections of Fig. 1. As a result of cell elongation, stalks bend towards the light, as shown in Fig. 1 (fourth section). Sunflowers are one of several plant species that make optimum use of light to increase growth rate.
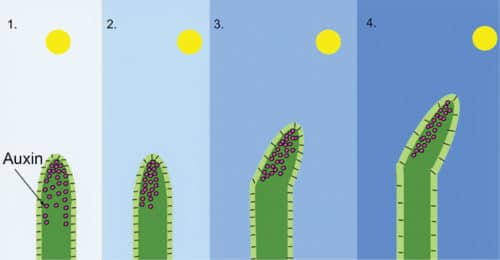
Mimicking this natural phenomenon, scientists have now built artificial stems with special materials to support solar cells that can track sunlight, like sunflowers. These stem-like artificial materials can bend towards light, are self-regulating and move into the optimal position needed to absorb Sun’s rays. Then, they make small adjustments to stay there as the Sun shifts. This allows them to capture about ninety per cent of available light shining on solar cells at a 75° angle.
Without tracking the Sun, loss can be enormous when incident angle is large (for example, 75 per cent loss at 75° incidence). These phototropic systems are termed as SunBOT, or sunflower-like biometric omnidirectional trackers, as shown in Fig. 2. These can autonomously and instantaneously detect and track incident light in 3D space at a broad range of ambient temperatures with high accuracy and fast response, without auxiliary power supply or human intervention. It has been demonstrated that artificial phototropism can achieve up to 400 per cent energy-harvesting enhancement over conventional non-tropistic materials under oblique illumination.
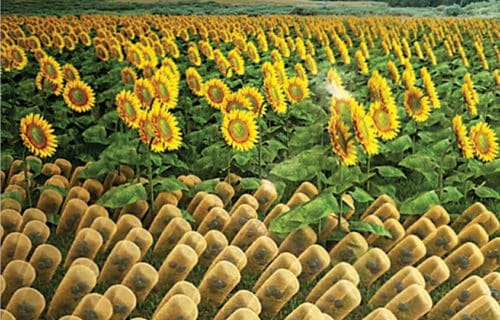
Importance of solar energy
Solar cells turn sunlight into electricity. This technology offers a more Earth-friendly way to produce power than burning coal and other fossil fuels. The importance of solar energy has increased every year. World population and energy needs have increased linearly. At present, total energy requirement of the world is about thirteen terawatt (TW). The total renewable energy source of the world under various heads is as follows: 0.5TW hydroelectric energy, 2TW to 4TW wind energy, 12TW geothermal energy, 2TW ocean wave energy and a stunning 120,000TW solar energy. This means we must tap this source efficiently for our increasing energy demands.
Present-day commercial silicon solar cells have about 22 per cent efficiency. One of the most efficient ways to increase photovoltaic performance is integrating heliotropism, that is, the ability to track the Sun across the sky, using solar cells for more efficient photon collection. Most active heliotropic systems that are used with solar panels have external positioning panels with motors, illuminance sensors, microcontroller CPUs, GPS tracking devices, etc. These systems are costly and need regular maintenance and power supply for operation.
The block diagram of an active heliotropic system is shown in Fig. 3. Integrating heliotropic property into the photovoltaic system renders the system passive, reducing extraneous parts. No external power supply is required because it is light-driven. It is very cost-effective and requires minimum maintenance.
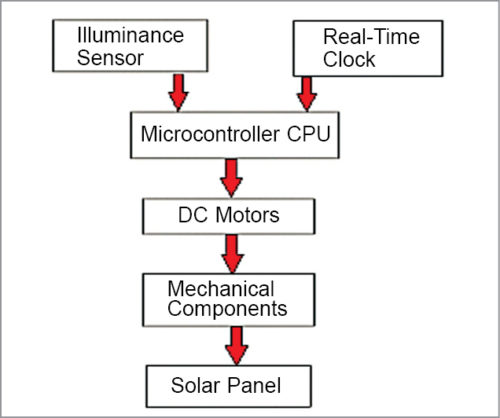
Design and operating principle of SunBOT
To mimic biological asymmetric growth, reversible photo-thermo-responsive soft materials that shrink on illumination are used to fabricate artificial stem-like cylindrical pillars. Like stems, SunBOT pillars bend towards stimuli due to asymmetric deformation between the illuminated high-temperature region and the shaded low-temperature region.
SunBOT can be constructed from many reversible thermo-responsive soft polymer materials like hydrogels, liquid crystal elastomers (LCEs) and azobenzene/spiropyran for mechanical actuation. Photo-thermally-responsive SunBOT materials like hydrogels are mixed homogeneously with nano-photo-absorbers, either gold nanoparticles or reduced graphene-oxide, which are all photo-responsive. The homogeneous mixture is packed in a polyurethane mesh for structural support.
These nanoparticles absorb light via plasmonic resonance modes and generate heat that changes volume of the thermo-responsive polymer in response to temperature change. The system has a low actuation temperature of 32°C. Reaction time is less than ten seconds and is tunable. One of the thermo-responsive smart hydrogels that is used often along with photo-responsive nanomaterial for construction of SunBOT is poly (N-isopropylacrylamide), that is, PNIPAAm, to create a system with tunable response time by varying concentration of nanoparticles.
Similar to sunflower, the photo-tracking process involves four steps: detection, actuation, aiming and recovery, as shown in Fig. 4. On illumination, SunBOT detects incident light via surface plasmon resonance of the incorporated nano-photo-absorbers and actuates towards the source due to local heating and asymmetric deformation.
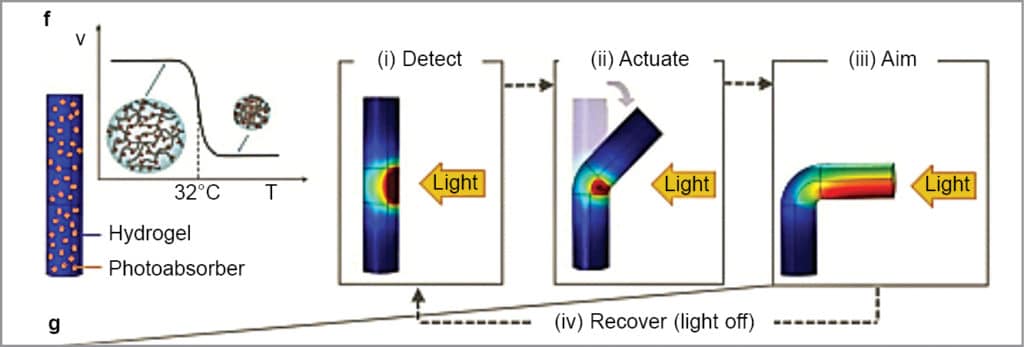
Asymmetric deformation is induced by establishing an appropriate temperature gradient across the pillar, where illuminated front-side temperature is more than the hydrogel’s lower critical solution temperature (LCST) and the shaded back-side temperature (Tb) remains below LCST. Hence, illuminated side shrinks.
This illuminated side shrinkage results in a bending effect similar to that observed in plant stem, as shown in Fig. 5. The figure also shows temperature distribution and stretch field of SunBOT at steady aiming state. As soon as SunBOT aims at the light source, it spontaneously terminates actuating motion. Aiming as a self-regulated actuation is modulated by the negative feedback loop inherent in tight hydrogel-stimuli interactions. Supporting an arrangement of solar cells with SunBOT stem is shown in Fig. 6.
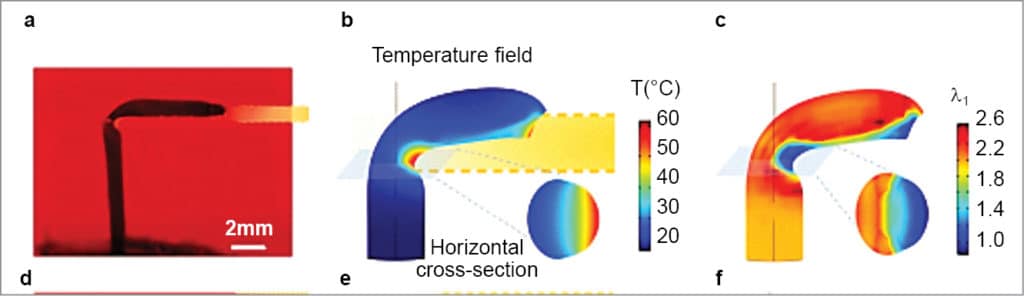
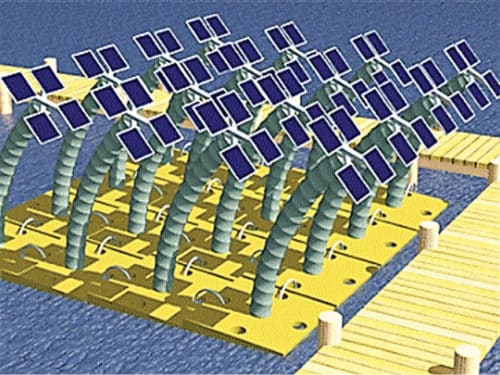
Smart hydrogels
Polymers are long chains of chemically-linked repeat units called monomers. Hydrogels are binary polymers, and are capable of absorbing and retaining water up to twenty times their dry weight. These are flexible, too.
Hydrogels can be conventional or stimulus-responsive. Stimulus-responsive hydrogels are called smart hydrogels and rapidly change their equilibrium state in response to external stimuli such as pH, temperature, electric field, magnetic field, light, pressure and so on.
Smart hydrogels responsive to heat are called thermo-responsive hydrogels, and solubility of polymer changes with temperature. Lower and upper boundaries of solubility are called LCST and upper critical solution temperature (UCST), respectively.
Plasmon resonance
Nanoparticles of noble metal, graphene, etc, whose sizes are comparable to incident light wavelength, interact with light by Mie scattering. Mie scattering suggests situations where size of the scattering particles is comparable to wavelength of the light, rather than much smaller or much larger.
Mie scattering arises when an electromagnetic wave incident on the particles and all ranges of wavelengths get reflected back equally to the atmosphere. Collective oscillations of free surface electrons are called plasmon. Nanoparticles of noble metal and graphene support surface plasmons, that is, oscillations of the conduction electrons at the nanoparticle surface that result in extraordinary optical properties that are not exhibited by any other class of material.
Light absorbed by the particles excites the free electrons. Specific wavelengths of light can drive conduction electrons in the nanoparticles having size around resonance frequency of light, to collectively oscillate—a phenomenon known as surface plasmon resonance (SPR).
When these resonances are excited, absorption and scattering intensities can be up to forty times higher than identically-sized other nanoparticles that are not plasmonic.
The photo-responsive nanoparticles of gold/graphene absorb light and generate heat through plasmon resonance, which is transferred to the surrounding hydrogel. When heat generated raises the temperature more than LCST of the smart hydrogel, the hydrogel starts to shrink. Bending of SunBOT stem is achieved by differential shrinking across the cross-section. The part closest to the light shrinks the most, while the part away from the light shrinks the least.
Tracking performance
Stimulated thermal and stretch fields within SunBOT pillars reveal that this wide-angle tracking ability is induced primarily by contraction at the illuminated region rather than governed by the material stiffness or flexural modulus, as in a typical elastic beam bending. This allows the freedom in material selection.
Further, phototropism of SunBOT is energy-efficient. As this light-powered hydrogel configuration is essentially a volumetric phase transition with a tunable LCST, it consumes a minute amount of photonic power to locally heat the hydrogel and trigger the release of a large amount of mechanical energy for phase transition.
SunBOT has a truly broad and tunable operation window that can guarantee successful phototropism over a wide range of light intensities, ambient temperatures, lighting conditions, irregular geometric shapes and rhythms of illumination. These can be achieved by tuning material properties, nano-photo-absorber concentration, transition temperature, dimensions of the material, etc. It can not only track a spotlight but also large area lighting.
The hydrogel has a fast recovery when not being illuminated. It can successfully track a light source in real time, continuously moving 360 degrees azimuthally at a fast speed up to 0.03 second per degree, and can precisely stop at the light source direction without over- or under-bending. An array of SunBOTS can, in principle, achieve up to 400 per cent solar energy, harvesting enhancement over non-tropistic materials at oblique illumination angles.
Other concepts of heliotropic solar cell
There are other variants of passive heliotropic and phototropic systems developed by several research institutes. In one system, six panels made from liquid crystalline elastomer for mechanical actuation, single wall carbon nanotubes for photo-thermal energy conversion and a polyeurethane mesh for structural support are assembled together to make the system. The elastomer, when heated above 68°C, changes its nematic order, leading to reversible axial contraction and mechanical actuation, enabling the system to be heliotropic.
Six composite structures made from these photo-thermal smart materials that concentrate light and act as an heat collector are bonded to six actuator beams that actuate (shrink) when incident sunlight increases temperature to about 68°C.
The above multi-stage design divides actuators into multiple units, each covering a certain angle. As the Sun moves during the day, one or two actuators that are facing the sun respond and pull the solar panel towards the Sun. These composite structures support a solar panel and move to track the Sun. This arrangement is shown in Fig. 7.
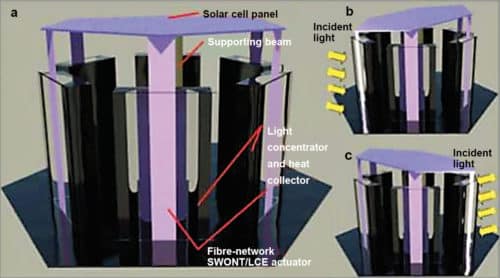
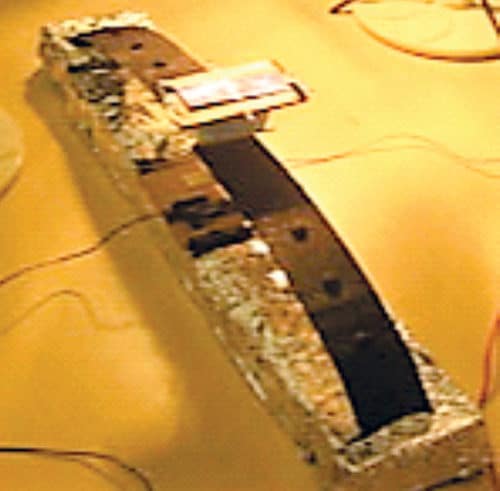
In another system, developed by Massachusetts Institute of Technology, solar panels are installed on top of a curved arch made of two kinds of metal, namely aluminium and steel. The mechanism relies on difference in coefficients of thermal expansion of the two metals. Expansion is caused by the temperature gradient between sunny and shaded areas. The light warming up one side causes the arch to bend, tilting the panels towards the light. This system has been reported to be maintenance-free. The arrangement is shown in Fig. 8.
Estimated cost
Presently, the monocrystalline silicon solar system with the ability to track the Sun, including storage battery, electrics and control system in line with the design shown in Fig. 3, is sold at about US$ 10/W (about ₹ 720/W), whereas a conventional monocrystalline solar system without tracking device but with storage battery and electrics costs about ₹ 70,000/kW, that is, ₹ 70/W. It is estimated that cost of SunBOT solar panels with electrics, when mass manufactured, will be compatible with conventional units. This is because there is no need to provide costly GPS tracking mechanism—instead, cost-effective smart polymers would be used. Further, it would change the scenario of green power generation as these smart devices would produce about forty per cent more electricity compared to conventional solar roof installations.
Rathindra Nath Biswas is a 1964-batch chemical engineering graduate from Jadavpur University, Calcutta. He was awarded a certificate for designing Benzol Plant by Giprokoks, USSR, and Certificate of Honour by Indian Institute of Metals. He has published 35 research papers in various journals. He retired from service as head – MECON, Durgapur.
Sunflowers only face east. They do not follow the sun all day, so if you’ve lost your directions, look for some sunflowers. They will be facing east.
Hi Robert, these SubBots are made up of Solar cells which looks like sunflowers.