A groundbreaking discovery paving the way for high-speed data transmission beneath the waves, eliminating the limitations of acoustic methods!
Oceans, covering more than 70% of the Earth’s surface, are often referred to as the last frontier of exploration. The underwater world is a realm of breathtaking beauty, wonder, and mystery. It is a world of vibrant marine life, ancient shipwrecks, and unexplored depths. Whether it is the depths of the Mariana Trench or the vibrant coral reefs of the Great Barrier Reef, our fascination with the underwater world knows no bounds. But beneath the serene surface, there is a challenging environment for communication.
Starting from strategic military applications to oil and gas exploration using remotely operated vehicles (ROVs) and autonomous underwater vehicles (AUVs), underwater tourism and marine life exploration require reliable and efficient communication systems. Traditional methods like acoustic communication have limitations, especially when it comes to data transfer rates and bandwidth.
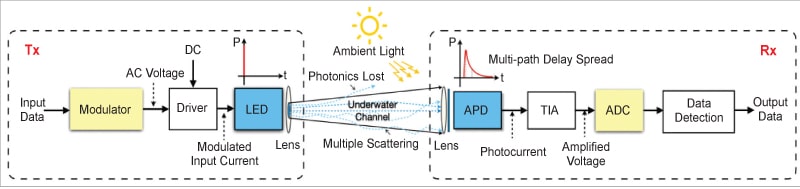
This is where underwater optical communication (UWOC) comes to the fore, providing high data rates, low latency, security, and reliability. At its core, underwater optical communication harnesses the principles of light propagation and modulation to encode and transmit information.
The architecture of the UWOC system
The main components of an underwater communication system include a transmitter, an underwater channel as the medium for light propagation, and a receiver as depicted in Fig. 1.
Transmitter
The transmitter module consists of a modulator based on the type of application being used and design complexity to modulate the properties of the input optical signal, an optical driver that amplifies, shapes, and controls electrical signals to drive optical components like laser diodes for efficient data transmission in optical communication systems, a light source (LED/laser depending upon the transmission depth), and a lens to focus the modulated optical signal towards the receiver.
Channel
Underwater channels can be broadly classified into four different categories based on different concentrations of dissolved particles, namely pure sea water, clear ocean water, coastal ocean water, and turbid harbour water.
Receiver
The receiver module consists of a lens to collect incident optical signals from the transmitter, an avalanche photo-diode, for capturing the optical signals and converting them into photocurrent, a trans-impedance amplifier to convert photocurrent into voltage values, an analogue to digital converter to convert analogue voltage into digital signals for data detection, and a data detection module to retrieve the transmitted data corrupted by the channel noise and detector imperfections.
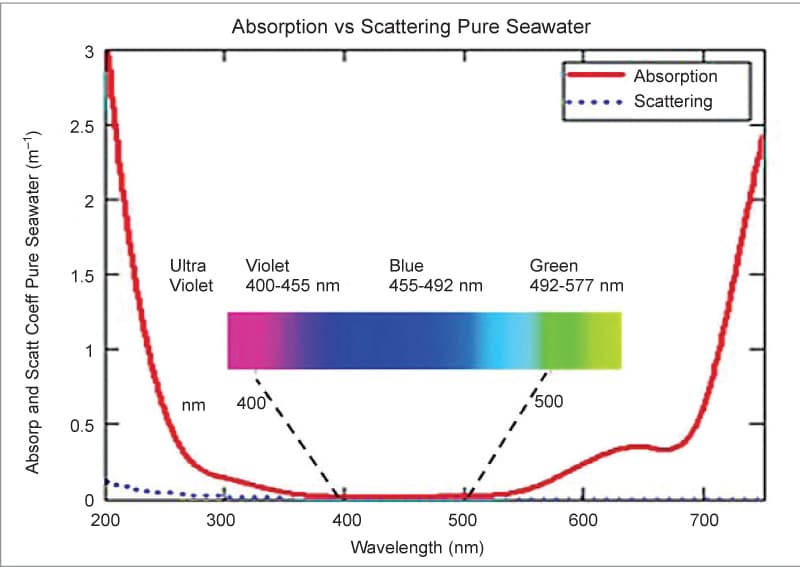
Factors affecting underwater optical communication
Some major factors that affect the underwater optical propagation are:
Water type
We encounter various types of water, each with its unique characteristics. These water types include pure seawater, transparent ocean water, coastal ocean water, and murky harbour water. Each of these water types exhibits distinct physical properties, including differences in temperature, impurity levels, and salinity levels.
Absorption and scattering
The attenuation of the optical signal in the underwater channel is mostly contributed to by absorption and scattering in the water. The beam extinction coefficient, c, for the attenuation is given as c(λ)=a(λ)+b(λ).
where a is the absorption coefficient and b is the scattering coefficient. Both coefficients are dependent on the wavelength being used for communication.
Particles in water
Various dissolved salts, ground quartz, clay minerals, metal oxides, coloured dissolved organic matter and other organic matter such as viruses, colloids, bacteria, phytoplankton, zooplankton, and organic detritus also affect the optical signal propagation in the water.
Turbulence
It can create signal attenuation, beam spread, signal distortion, modal noise, and signal disruption in underwater optical communication.
Pointing and alignment
Pure seawater requires tight pointing requirements as the optical beam is likely to follow a collimated path. On the other hand, in turbid harbour, scattering is the significant contributor causing the optical beam to spread in different directions. A misalignment of three degrees reduces the detector response by a factor of hundred.
Background noise
Scattered light collected by the receiver, background noise from the Sun, and diffused extended background noise affect the UWOC.
Wavelength used in UWOC system
Fig. 2 shows the absorption and scattering of various wavelengths in pure seawater. The bandwidth used in UWOC is typically 400-500nm due to their minimum attenuation (low absorption and low scattering), which is a key driver of high data rate and longer distance. Most of the undergoing projects are working at this band for the UWOC system.
Advantages of UWOC
The UWOC has several advantages over traditional radio and acoustic communication systems. Some key advantages are:
High data rates
One of the significant advantages of underwater optical communication is its high data transfer rates. It can transmit data at rates several times faster than traditional acoustic communication (up to Gbps data rates over a distance of tens of metres).
Low latency
Optical signals travel at the speed of light, which results in lower latency compared to acoustic signals.
Low interference
Unlike acoustic communication, optical communication is not affected by underwater noise from marine life or other sources.
Secure
Laser signals are highly directional, making it difficult for eavesdroppers to intercept the communication.
Less environmental impact
Optical communication does not produce the same level of noise pollution as acoustic communication, which can be disruptive to marine life. It is a more environmentally friendly option in this regard.
Applications
The potential applications of underwater optical communication are vast and include:
Subsea exploration
Oceanographers, marine biologists, and other scientists often use underwater optical communication to transmit data from sensors, cameras, and other instruments on underwater vehicles, remotely operated vehicles, and autonomous underwater vehicles. This enables real-time monitoring and data collection from deep-sea environments.
Environmental monitoring
Underwater optical communication is employed for environmental monitoring, including the measurement of water quality, temperature, and other parameters in oceans, lakes, and rivers. Transmitting this data in real time is important for assessing environmental conditions.
Military and defence
Underwater optical communication is employed by the military for secure and high-bandwidth communication between submarines, divers, and surface vessels. It helps maintain situational awareness and supports various naval operations.
Underwater infrastructure
In the oil and gas industry, underwater optical communication is used for tasks such as controlling remotely operated vehicles and transmitting data between underwater sensors and surface facilities. This is crucial for the maintenance and operation of subsea infrastructure.
Underwater imaging and surveillance
Optical communication is used in underwater imaging and surveillance systems to transmit high-definition video and images in real time. This is valuable for underwater security, search and rescue operations, and underwater archaeology.
Standardisation activities
Standard development organisations like and International Electrotechnical Commission (IEC), Institute of Electrical and Electronics Engineers (IEEE), and International Telecommunication Union (ITU) are working on various aspects of UWOC.
IEC 62628
This standard provides guidelines and requirements for optical wireless communication systems used in underwater applications, including communication between underwater vehicles, sensors, and other devices. It covers aspects such as modulation schemes, transmission protocols, and system performance.
IEEE 802.15.7
This standard focuses on visible light communication systems, which can be adapted for underwater optical communication. It defines the physical layer and media access control layer specifications for short-range optical wireless communication.
ITU-T G.6980
While not specifically designed for underwater communication, this ITU standard defines the grid for wavelength division multiplexing applications. Some underwater optical communication systems may leverage wavelength division multiplexing technology for increased data rates and channel capacity.
Challenges in UWOC
UWOC is a growing technology with boundless potential. Yet, with this growth comes a set of challenges:
The range limitations
Water, the very medium we seek to communicate through, poses a formidable challenge. Its tendency to absorb and scatter light limits the range of underwater optical communication systems. Researchers are tirelessly working on extending these limits through signal processing advancements and innovative beam-forming techniques.
Murky waters
The clarity of water varies significantly, and impurities and turbidity can cloud optical signals. Adaptable and robust signal processing algorithms are vital to combat these environmental factors.
The dance of precision
Maintaining the delicate alignment between transmitting and receiving devices in dynamic underwater environments is akin to a ballet. The constant motion of water necessitates sophisticated tracking and beam-forming systems.
The cost of exploration
Implementing underwater optical communication can be expensive, especially for deep-sea applications. Balancing the costs while pushing the boundaries of technology requires judicious investment.
Bioluminescence and ambient light
Bioluminescence and ambient light present significant obstacles for UWOC systems in their efforts to enhance data transmission. Bioluminescence is the capacity of specific organisms to generate light via a chemical process. Ambient light arises from sunlight scattering and bending as it passes through the ocean’s layers.
Underwater optical communication represents a transformative technology for exploring and exploiting the depths of our oceans. Its high data rates, low latency, and secure transmission open up new possibilities for underwater research, exploration, and applications. As researchers continue to overcome the challenges associated with this technology, we can expect to see even more innovative applications in the future, shedding light on the mysteries of the deep.
Suryansh Pratap Singh works as Research Engineer at the Centre for Development of Telematics (C-DOT), the telecom R&D centre of the government of India. He holds a B.Tech degree in electronics and communication engineering from MNNIT Allahabad
Arka Mukherjee works as Team Leader at the Centre for Development of Telematics (C-DOT), the telecom R&D centre of the government of India. He has more than eleven years of experience in embedded systems design for optical access networks and quantum key distribution systems