War veterans return home with all kinds of injuries—some physical, others psychological. A missing limb, an ear blown off, scars of wars can be seen all over. Advances in technology that could fill out missing pieces sound good, do they not?
Researchers at Wake Forest Institute for Regenerative Medicine at Wake Forest Baptist Medical Center, USA, have custom-designed a 3D printer and successfully printed ear, bone and muscle structures to take care of just that. The developed structures have been implanted in animals and matured into functional tissue, apart from developing a system of blood vessels. Probably the future does hold an all-perfect world. Let us take a look at how they do it.
Printing body parts
In a typical plastic modelling 3D printer, basics would involve melting down the material and shaping it back in the form of the required design. However, biological tissue would not be suited to a similar setup and could be easily destroyed. Additionally, a support system would be required in place for the biomaterial to hold onto—similar to the support system in a traditional 3D printer.
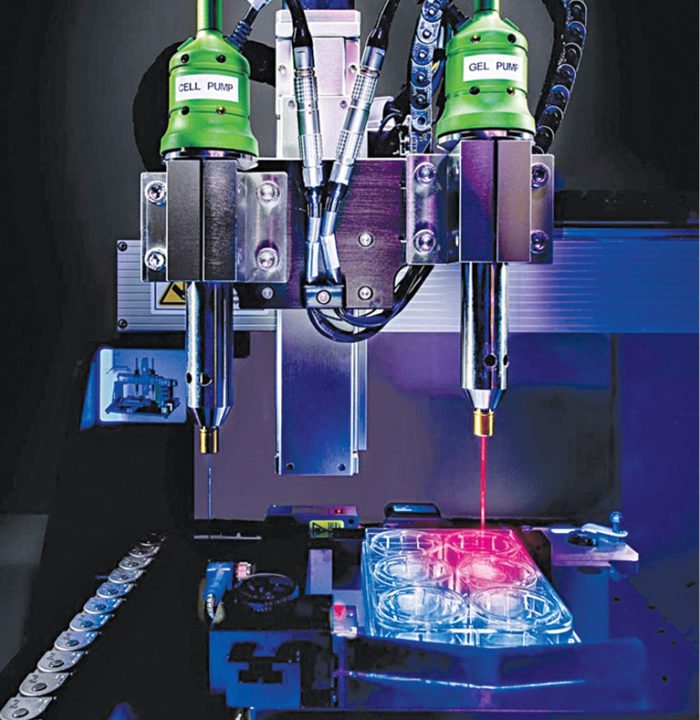
In addition to outputting cells, most bio-printers also output a dissolvable gel to support and protect the cells during printing. Organovo’s bio-printing process centres around identification of architectural and compositional elements of a target tissue, and creation of a design that can be utilised by a bio-printer to generate that tissue in the laboratory environment.
Once a tissue design is established, the first step is to develop the bioprocess protocols required to generate multi-cellular building blocks from cells that would be used to build the target tissue.
According to Organovo, the bio-printing process can be tailored to produce tissues in a variety of formats. Ranging from micro-scale tissues contained in standard multi-well tissue culture plates to larger structures suitable for placement onto bioreactors for biomechanical conditioning.
Complex organs are difficult to print
“The simplest organ is skin, which is a flat structure. As organs move up the ladder of complexity, from tubular structures such as blood vessels, hollow organs like the bladder and stomach, and solid organs like the kidney and liver, these require multiple cell types and more oxygen because these are dense with cells. Being able to meet the oxygen requirements of solid organs is a major challenge,” explained the bio-printing team at Wake Forest Institute for Regenerative Medicine.
Materials involved in casting
Scaffold materials involved in designing have to be biocompatible, of suitable strength and have shaping characteristics. The materials being used currently are selected either due to their compatibility with cell growth and function or due to their cross-linking or extrusion characteristics. Fibrin hydrogel materials and alginate-like polymers are mostly being used in printing. Other naturally-derived polymers include gelatin, collagen, fibrin, chitosan and hyaluronic acid.
Some synthetic polymers include polyethylene glycol, polycaprolactone, polylactic acid, polyglycolic acid and poly (lactic-co-glycolic) acid. A 3D extracellular matrix helps in preserving the native tissue architecture.
The machine that prints humans
The typical system, known as integrated tissue and organ printing system (ITOP), was developed into the current 3D bio-printer we have today over a period of ten years. The technology used in the development of bio-printers is another interesting one. The idea to heal the most difficult of wounds came from inkjet printers.
Experts from Wake Forest Institute explain the schematics of the machine. Multiple cartridges are used to deliver and pattern multiple cell-laden composite hydrogels, supporting thermoplastic polymer and a sacrificial removable hydrogel. End of each cartridge is connected to a micro-scale nozzle, and the top is connected to an air-pressure controller for precisely controlling the dispensing volume.
A heating unit ensures that the melted polymer remains easily dispensable. A three-axis motorised stage system enables 3D patterning of multiple cells and bio-absorbable materials.
The system resides in a humidified and temperature-controlled enclosure with clean environment. It works by depositing the hydrogel that contains cells, along with the scaffold material.
The challenge here is to ensure the life of the tissue structure is long enough for successful integration with the human body. This issue is addressed by a lattice structure of micro-channels that allow nutrients and oxygen from the body to pass into the graft until the tissue regenerates its own blood vessels.
Growth in systems
Dispensing based 3D printing has been suggested for direct 3D cell printing of cell-laden hydrogel constructs with a larger volume. Although this system generally has low resolution for making a cell pattern, dispensing of hydrogel solution with a high flow rate allows more rapid printing of 3D cell-laden hydrogel constructs. Major developments in the ITOP system have been related to nozzles and dispensing modules that can print at a higher resolution.
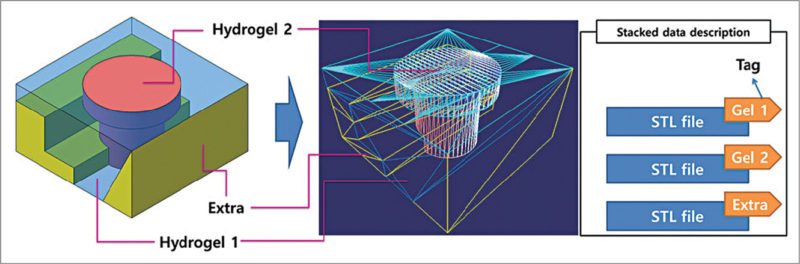
Researchers at Pohang University of Science and Technology (POSTECH) in South Korea have reported a computer aided design (CAD) and manufacturing system for multiple-head 3D printing, and have printed heterogeneous tissue models using two kinds of cell-laden hydrogel.
CATIA V5, a 3D CAD design software from Dassault Systemes, is being used by researchers at POSTECH in their design process. A paper published by Jin Woo Jung and Jung-Seob Lee, researchers at Department of Mechanical Engineering, POSTECH, explained the use of CATIA V5 for designing the exterior structure of heterogeneous tissues. The resulting models had two main tissue parts, plus a support part.
3D free-form structures are printed via vertical stacking of 2D patterns generated from cross-sections of the designed 3D model. The slicing algorithm involves calculating contours of the cross-sections of successive planes. Printing path for the frame is defined based on cross-section contours of the two cell-laden hydrogel parts.
Scaffold-free printing is here
Scaffold-free printing is another area that is catching up in recent years. Dr Gabor Forgacs, founder of Organovo and Modern Meadow, with his academic partners, leveraged the understanding of mammalian cells, to come up with another interesting system. They were able to demonstrate directed assembly of 3D tissues without dependency on integrated scaffolding or biomaterial components.
Similar results have been published in a paper on scaffold-free tubular tissues created by a bio-3D printer, co-authored by K. Nakayama, co-founder Cyfuse Biomedical. Cyfuse Biomedical K.K. is a Japanese corporation involved in development, manufacturing and sale of 3D bio-printers.
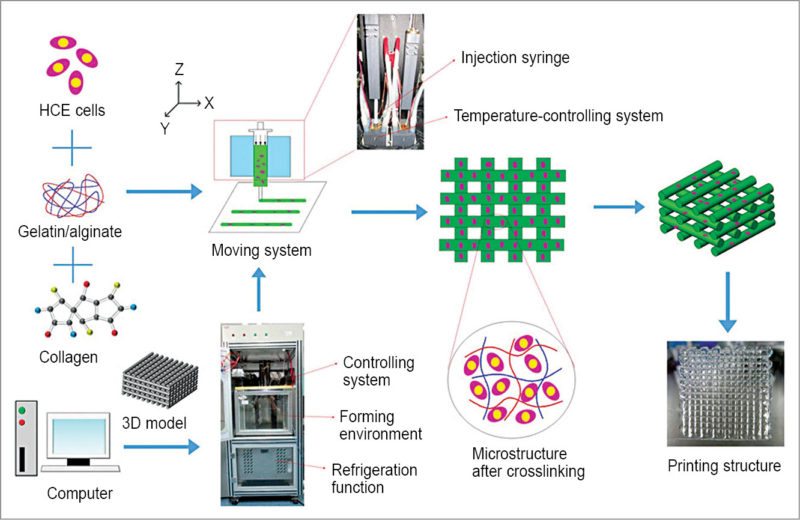
There is always room for development
Printing a tissue, at present, takes about two months. Much of the six to eight weeks required to develop a tissue or organ is dedicated to expanding the patient’s cells in the laboratory. Only a small percentage of the time is required for the printing process. So a method to speed up this process would be to print these directly into the human body.
However, there is still some time before we reach that stage. Rapid-curing bio-ink and 3D-scanning technology to detect a wound region would be a good place to start though. Additionally, with features to reverse-engineer medical images to CAD models, we could very well see some advances in in-situ printing.
The eventual long-term goal for bio-printing is to produce full organs. At present an average-sized lever takes days to manufacture. This is a very slow rate when we look at the number of people on the transplant list.
How does healing wounds in real-time sound? Imagine going to sleep with a battered body but waking up physically healed. Sounds like something straight out of Star Trek, does it not? A bit far-fetched, may be?
Saurabh Durgapal is working as technology journalist at EFY