The power output of these diamond batteries is low as compared to other alternatives. However, their total longevity favours them to become critical components of future electronics manufacturing.
Innovation in the area of batteries has attracted considerable attention from the scientific research community, given the need for greater energy efficiency and low-carbon techniques to supply clean power to sustain the energy evolution. With alternate, sustainable, natural, and renewable sources of energy being sought after, there is a deep interest in a nuclear-powered battery that generates electricity using nuclear waste.
A team of scientists from the University of Bristol in the UK has grown an artificial diamond in the lab. When placed in a radioactive radiation field, it generates a small electrical current. This development is expected to provide solutions for better management of nuclear waste and make clean electricity available.
The scientists hope to recycle waste from decommissioned nuclear power plants. Their work remains confidential, and not much information is available in public. Unlike most of the electricity-generation technologies that use energy to move a magnet through a coil of wire to generate a current, the man-made radioactive diamond produces electric charge simply by placing it near a radioactive source. Carbon-14 or C-14 is created in graphite rods, which are used in power plants to moderate the nuclear reaction.
The radioactive C-14 gets concentrated at the surface of these blocks, from where it is extracted and fused into a diamond to produce a nuclear-powered diamond. This is encapsulated in an artificial diamond to ensure safe containment of the radiation. The lifetime of diamond batteries far exceeds the life of conventional batteries as the half-life of C-14 is as many as 5,730 years. Diamond batteries, which are highly durable, provide charge for thousands of years and serve well as a low-voltage energy source. There are several low-voltage devices like pacemakers, satellites, and space probe components that cannot be easily accessed for replacement of their batteries.

One of the serious problems of using nuclear energy in power plants is the management of nuclear waste that remains radioactive for tens of thousands of years. This exciting technology holds great promise as large amounts of nuclear waste can be converted into a usable electricity source.
How it all started?
Materials engineering professor Dr Tom Scott’s research at the University of Bristol is based on understanding the basic and changing properties of materials in various engineered and natural environments to better forecast their performance and create methods for enhancing them. He has spent a considerable amount of time researching for and on behalf of the nuclear industry. He is the technical director of spin-out ImiTec Ltd, a company specialised in the detection and mapping of radioactive materials in industrial, urban, and environmental settings.
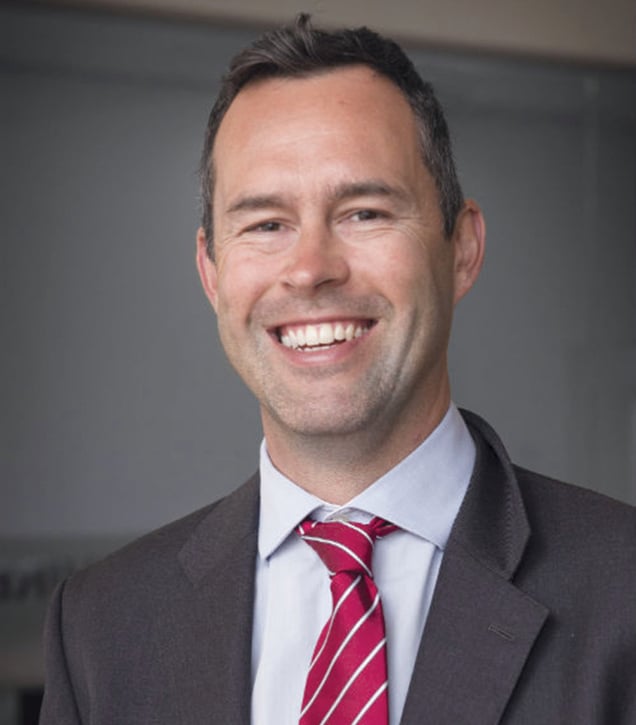
In his talk ‘Diamonds Are Forever,’ the professor opined that we are solving two problems in one shot: the disposal of the build-up of radioactive graphite in spent nuclear fuel (SNF) and the need for a long-lasting source of constant energy.
The first-generation reactors used graphite blocks as moderators to slow down neutrons to keep the nuclear fission process running. Instead of burying radioactive C-14 formed on the surface of graphite, Scott suggested extracting and using it for creating ‘deliberately radioactive’ diamonds, which when encapsulated inside an artificial diamond could produce a nuclear-powered battery for a long-term supply of clean energy.
He explained that extracting radioactive C-14 can also provide a practical solution to the problem of nuclear waste disposal. This technique was first demonstrated by the researchers in 2016 when they grew a man-made radioactive diamond in the lab, which was placed into a radioactive field to generate a small electric current.
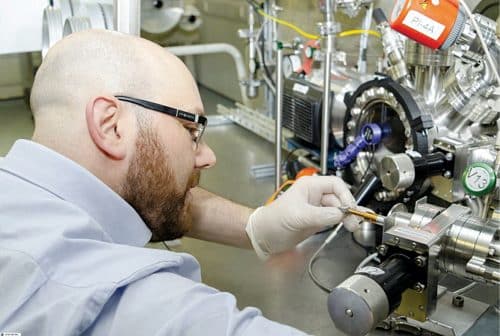
Scientists at the UK Atomic Energy Authority’s (UKAEA’s) Hydrogen-3 Advanced Technology facility (H3AT) are now working on a pilot project to establish a production line for the batteries capable of the initial supply of up to 20,000 devices per year.
Producing radioactive diamonds
Natural diamonds, made up entirely of carbon-12, take hundreds of thousands to millions of years to form naturally when subject to high pressures deep in the earth’s crust. These do not contain any radioactive carbon. Artificial diamonds are produced using a good carbon source such as graphite and a machine to create high pressure. However, these do not contain any radioactive material. Radioactive man-made diamonds for usage in diamond batteries are made from radioactive C-14 isotopes, which are known to be the largest nuclear waste by volume.
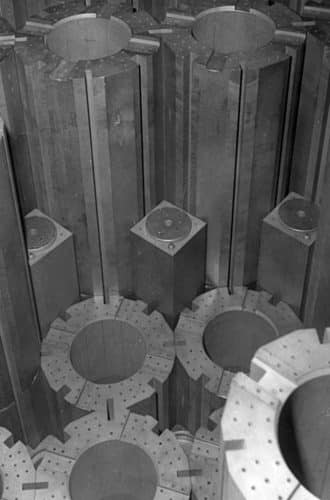
In nuclear power reactors, fissile uranium rods are placed inside graphite blocks that act as neutron moderators to slow down the fast-moving neutrons. During the nuclear fission, uranium atoms get split, generating heat that vaporises water into steam, which turns electricity-generating turbines.
During the process, some of the non-radioactive C-12 and C-13 isotopes in graphite blocks capture neutrons and get converted into radioactive C-14, which mainly concentrates on the outer parts of the blocks and can be effectively removed by heating them to the sublimation point of 3,642 degrees centigrade.
This results in releasing C-14 in gaseous form, and disposal of the less radioactive graphite blocks also becomes easier. C-14 gas is collected and subject to low pressure and elevated temperature to produce artificial radioactive diamonds using a process called chemical vapour deposition (CVD).
The diamonds are encased in non-radioactive artificial diamonds that provide protection by containing the radiation. It is highly dangerous to ingest or touch radioactive diamonds with naked skin, but they remain safe when encapsulated as no short-range radiation can escape. Being the hardest substance known, diamonds offer the best protection. Scott and his team have managed to create a prototype using a blend of C-14 and bits of tritium (a form of radioactive hydrogen) as the source of beta-radiation.
How diamond batteries work
Functionally, the concept of a diamond battery is similar to that of radioisotope electric generators used for space probes and rovers. The diamond battery generates electric charge through direct energy conversion using a semiconductor diode and C-14 as a radioisotope source. C-14, while undergoing decay, emits short-range low-energy beta particles (essentially the nucleus’ version of electrons) and turns into nitrogen-14, which is not radioactive and gets absorbed in the diamond casing.

The beta particles released by C-14, moving with an average energy of 50keV, undergo inelastic collisions with other carbon atoms present in the diamond structure, causing electrons to jump from the valence band to the conduction band, leaving behind holes in the valence band where electrons were earlier present. Successive electron-hole pairs get collected at the metal contact attached to the diamond. C-14 is preferred as the source material because its beta particle radiation is easily absorbed by any solid.
Some devices use tritium and beryllium along with C-14 as additional beta-emitting radioisotopes. The amount of C-14 used in each battery is yet to be disclosed, but what is known is that a battery containing 1gm of C-14 would deliver 15 joules (15J) per day, much less than an AA battery, and would take 5,730 years to reach fifty per cent power.
Alkaline AA batteries are designed for short timeframe discharge, and one battery weighing about 20gm has an energy storage rating of 700J/gm. When operated continuously, it would run out in 24 hours.
Diamond batteries do not provide power directly to attached devices but are designed with a capacitor that gets ‘trickle charged’ by the battery and then discharges to power the devices at set intervals. The following characteristics are common for a prototype:
Voltage: 2V (estimated)
Energy: 31MJ over first 5,700 years
Prototype size: 10mm×10mm× 0.5mm (plus electrodes)
Temperature: Physically stable at 750 degrees Celsius
Powering the future-nuclear batteries
Atomic battery, nuclear battery, tritium battery, or radioisotope generator are devices that use energy from the decay of radioactive isotopes to generate electric current. These differ from nuclear reactors and do not use a chain reaction. Nuclear batteries use radioactivity to generate power instead of storing a certain amount of charge.
Compared to chemical batteries, nuclear batteries are characterised by higher volumetric energy density (therefore longer battery life) and stronger endurance in harsh conditions. Nuclear batteries are used in space exploration applications. It is impossible or extremely costly to access a device once it has been launched into space, and solar energy is not a practical solution to powering electronic equipment since its availability is limited.
NASA uses nuclear battery technology called Radioactive Thermoelectric Generator (RTG) to power spacecraft. Batteries used for implantable medical devices (IMDs) need to function over a long period of time without being accessed for recharge or maintenance and should be limited in size and weight. Nuclear batteries called betavoltaic cells were widely used in IMDs during the 1970s; however, the potential risk of radiation led to the shift towards lithium-ion batteries in the 1980s. With the recent advancements in nuclear batteries, the use of betavoltaic cells is being reconsidered.

Radioisotope thermal generators
Radioisotope thermal generators (RTGs) use thermal energy of a radioisotope decay to heat a thermocouple, which produces infrared radiation to power ‘solar cells’ by generating several hundred milliwatts of electrical power.
A large amount of radioactive material is required for an effective RTG, restricting its application because of high radiation dangers. NASA has been using RTG for the past thirty years or so. The United States first launched a radioisotope thermoelectric generator (RTG) into space on a satellite in 1961. They have powered NASA’s twin Voyager probes right from the year 1977 when these were launched. Having covered more than sixteen billion kilometres, the Voyagers are the most distant spacecraft from Earth and are still travelling fine.
RTGs have enabled many other missions, such as NASA’s Mars Curiosity rover, to provide the communication links between the scientists and engineers on Earth and the missions in space. As RTGs are quite heavy, expensive, and inefficient, NASA is also experimenting with Stirling Engine patented by Robert Stirling in 1816.
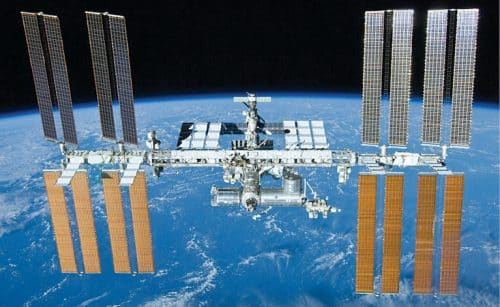
The Stirling engine consists of two chambers—cold and hot. It uses a ‘working fluid’ (commonly air, helium, or hydrogen) with a regenerator or heat exchanger between the two chambers. The difference in temperature and pressure between the two chambers causes the working fluid to expand and contract, resulting in a net conversion of thermal energy to mechanical energy.
Indirect conversion batteries
Indirect conversion batteries involve radioactive decay radiation consisting of alpha or beta particles impinging on radioluminescent material like phosphor to produce ultra-photons that are collected using photodiodes such as InAs, GaP, GaAs, and AlGaAs. The radioluminescent materials used are nanotubular or micro spherical structures filled with tritium thin films. The photons are guided to a remotely located photodiode. Waveguides are used to protect the diode from the radiation.
The overall efficiency of two per cent at 3.5V is possible if the spectra are matched, and power conversion is optimal. The battery life depends on the half-life of the radioisotope used as well as on the rate at which the radioluminescent material degrades, which many times turns out to be faster than the rate at which the radioactivity falls.
Betavoltaic cells
Betavoltaic cells consist of a beta-emitting source, such as Tritium, coupled to a p-n diode.
Energetic beta particles (typically a few keV) emitted by the source hit the semiconductor, producing hundreds of thousands of electron-hole pairs. Most of them get swept across the electric field in the p-n diode to produce current. Some losses occur as some of the excited electron-hole pairs undergo recombination, producing photon and/or phonon.
Since betavoltaic cells can operate in extreme environmental conditions, these have replaced conventional chemical batteries as power sources in many low-power applications such as micro-electromechanical and electronic devices, implantable biomedical prosthetic devices, and military intelligence applications.
Betavoltaic cells have low-power output and are not suitable for consumer electronics where energy requirement is comparatively higher. A battery 1cm-squared wide and 0.2cm tall generates only one microwatt of power, whereas a smartphone needs about 1,857mW.
Application areas
Nuclear batteries have several advantages over the traditional sources of power, making them useful for applications in several fields. These radioisotope batteries have a high energy density and can function over wide ranges of environmental conditions of temperature, pressure, underwater, or in space.
The batteries have been tested in places as extreme as the top of a volcano and deep under the sea. Since radioisotope decay is sustained by the material itself, there is no need for refuelling or recharging.
The disadvantage of using these batteries is that their power density is lower than the chemical batteries, and these have low conversion efficiencies.
These batteries would be highly useful in environments where conventional power sources cannot easily be replaced, such as implanted hearing aids, artificial pancreas, or pacemakers. No extension cord is long enough to take to another planet, and we know that there is no spacecraft charging station available during the journey as yet. That’s why researchers are working hard on ways to make spacecraft power systems more efficient, resilient, and long-lasting.
Due to a very long life, diamond nuclear batteries could power spacecraft or a satellite, increasing the flight time and enabling much faraway travel than what is currently possible.
A tiny, robust power source that does not require any mediator interface, wiring, or conductors but only a chemical reaction that generates a low, continuous electrical current has immense value in outer space.
The high energy capacity of nuclear batteries makes them suitable for applications ranging from environmental health monitoring to structural health monitoring. These are also used in a variety of industrial applications, including electron capture devices for gas chromatography. The technology has already been established in practice on a small scale at the University of Bristol.
One of the challenges now is to put in place an assembly line for these diamond batteries for large scale production as soon as possible. Another issue that needs to be tackled is regulatory acceptance and approval, that is, what is acceptable by regulatory bodies in different countries and what is not in terms of manufacturing such batteries for a mainstream consumer market.
Conclusion
The nuclear-based radioactive isotopes batteries involve no moving parts, zero maintenance, and just direct and continuous electricity generation.
The power output of these diamond batteries is low as compared to other alternatives. However, their total longevity favours them to become critical components of future electronics manufacturing. By encapsulating radioactive materials inside diamonds, it is possible to turn a long-term serious problem of nuclear waste management into a nuclear-powered battery for a long-term supply of clean energy.
A large number of graphite blocks are stored in various parts of the world. When Britain’s Magnox Nuclear reactors were withdrawn in 2015 after more than fifty years of service, the nuclear waste consisted of 95,000 tonnes of graphite blocks used as moderators to manage the nuclear fission process. This technology would make it possible to significantly reduce radioactivity and thus the cost of disposal.
Comparatively, the cost of producing an artificial diamond is much less than disposing of used nuclear fuel and nuclear waste. It is expected that by 2021, despite efforts, the US would have radioactive fuel, which is sufficient only for two and a half nuclear battery modules a year. However, the Mars rover known as ‘Curiosity’ itself required eight nuclear battery modules.
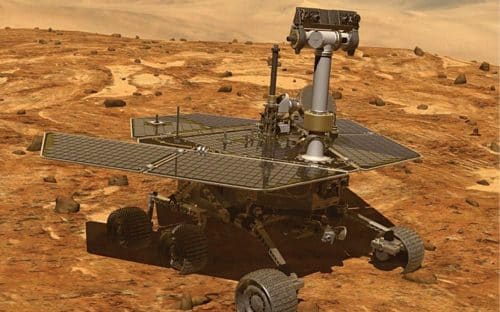
This scarcity and the small existing inventory are inadequate for the ambitious planetary missions planned till 2030 to destinations such as the very cold moons of Jupiter and Saturn. Hence, NASA has been examining and analysing alternatives. Continuous research and development are in progress for making safe, compact, high energy capacity, and long life batteries a reality for applications ranging from environmental, medical to structural health monitoring.
Dr Deepak Halan is associate professor at School of Management Sciences, Apeejay Stya University
What an absolute waste of time and effort. This is akin to solar roadways or Elon musks hyperloop!
Seriously why is this making news? Just a flying glimpse at the “non-science” behind this fairy tale process and you realise it’s absolute trash!! Shame on you electronicforu.
Check out “thunderfoot” on YouTube who explains why this is nonsense!!
We are very sorry to hear that you did not like the Technology used in this article. We always try to provide something new to our users. Some like it and some do not. Nevertheless, we accept your comment as a constructive feedback and will try to make our research even better and provide you better reading experience.
Thunderfoot is however debunking a completely different type of battery and it has nothing to do with the above article.
Have you actually read it?
could Nuclear Diamonds ( + Fusion ? ) be power boosted for PRIMARY power of Space Mining as well as Exploration
I thought it was a great piece and exciting technology. However, I’m shocked to hear there’s a shortage of potential fuel in the u.s… We hear the opposite, and the controversy of where to store spent nuclear waste.
Thank you for your valuable feedback.
Liked the article. Good and simple information. Became a little lengthy but good
So essentially this would compete with low-energy “power harvesting” solutions for small-load devices with intermittency? It sounds like the electronics between battery and device might even be identical.
Disposal of nuclear waste in such batteries does make sense. Probably it will open ways of making long lasting batteries in future.
This is the future. May be in 100 years we will power all electronic devices with lifetime batteries. Bravo,
Ivana TGTG
Nice little article – thank you!!
You are most welcome.
Just like my preposterous idea of using AI to help sustain regression automated testing, which was thought of someone else with enough willpower to actually make it happen (see testim) this is also one of my impossible ideas of having cristal-like (rhombohedron shaped) battery which would have power for a very long time. And again, it seems like it is possible. Nothing is nonsense anymore. Everything, no matter how exaggerated it would seem to be, can and will be used in the future. It doesn’t matter if it’s useless now, at the very least this could be used as a stepping stone for future enhancements or even new developments of technology. Keep on writing your articles. The only thing is, it would be great if you’d involve some scientific articles as references.
An excellent and very interesting article on nuclear battery technology. This is the future for battery technology so just ignore that previous dweeb’s comment as he has no idea what he is talking about and should go back to the ignorance hole he crawled out of!
Thank you for your valuable feedback.
I enjoyed the article. It would be nice though if this battery could be made more efficient. I suspect it can.
Thank you for your valuable feedback.
Or if you could increase output or overall power by using more at once?
My understanding of the thermionic generators were that the heat generated electricity in the thermo couple that was used directly.
I don’t see why the thermo couple would power an infra red source that would power a solar cell.
This would be quite an energy loss.
Perhaps there was an error in the article?
Pay no.attwntion to the grinch. I found this to be an interesting and informative article.
Thank you for your valuable feedback.
Curiosity — aka thinking outside the box has been the inspiration for more advancements in many areas than anyone can imagine. I found this article extremely interesting — at my age — I doubt if I will see it become highly used; however, knowing that a solution to radioactive storage problem is being addressed gives me hope that my great grandkids and beyond will have a better life. Thanks for the article and please never stop teaching and learning.
Thank you for your valuable feedback.
So is this kinda like a photovoltaic cell, but for beta radiation instead photons?
Please send me more information on diamold batteries