Read Part 5
Use of IEDs by terrorists have brought to the fore the need for stand-off detection and identification of chemical, biological and explosive agents.
The need and relevance of efficient and reliable technologies for stand-off detection and identification of chemical, biological and explosive agents have become increasingly important in present-day scenario. Chemical and biological weapons are relatively inexpensive to produce and can unleash a devastating effect as terrorist weapons.
The international community has already witnessed devastating effects these weapons can cause on more than one occasion. Millions have been affected by the destruction caused by these weapons of mass destruction in the past. Sarin attack by Aum Shinrikyo cult in 1995 in a Tokyo subway, hydrogen cyanide and mustard gas attack by Iraq in Anfal campaign against Kurds most notably in Halabja massacre in 1988 (Fig. 1) and international dispersal of anthrax spores against the US in 2001 are some examples of chemical and biological weapon attacks.
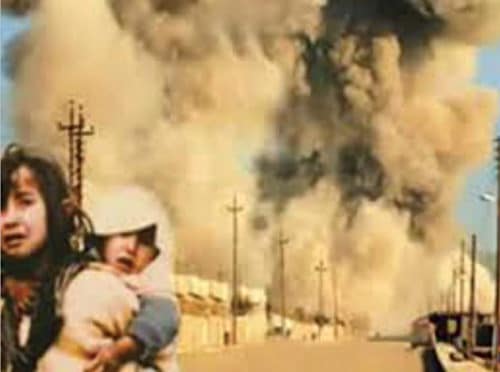
Use of improvised explosive devices (IEDs) by terrorists and anti-national elements killing soldiers and civilians alike has become a routine affair. The incidents have brought to the fore the need for stand-off detection and identification of chemical, biological and explosive agents. A number of laser-based methodologies/techniques are either in use or being explored for the purpose.
Detection of chemical warfare agents
Differential absorption lidar
Nerve agents (such as organophosphate compounds) and blister agents (such as mustard compounds), because of their acute toxicity, rapidity of action, indetectability by human senses and economic viability, are two potentially harmful classes of chemical warfare agents.
Nerve agents are organophosphoris easters, which initially stimulate and then paralyse certain nerve transmissions by interfering with cholinesterase enzymes. Common chemical warfare agents belonging to this category are tabun, sarin, soman, Vx, dichlorvos and malathion. Fig. 2 shows typical effects of a chemical warfare agent attack.
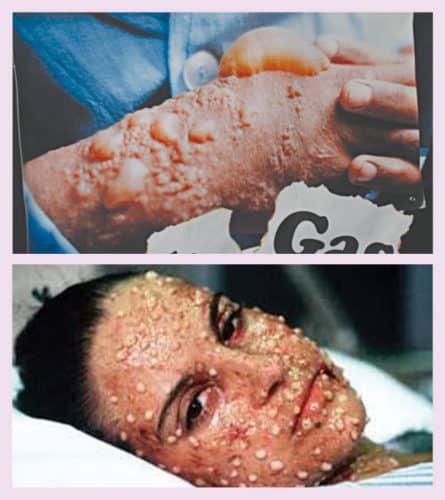
Lidar-based detection is the most practically realisable technique for stand-off detection of chemical and biological warfare agents. Commonly exploited physical phenomena forming the basis of chemical and biological agent detection include elastic backscattering, laser-induced fluorescence (LIF) and differential absorption.
These are superior to point detection systems such as infrared (IR) spectroscopy and Raman spectroscopy. This is due to their capability to range and discriminate chemical and biological molecules in real time.
Ultraviolet (UV) LIF can be used for the detection of biological molecules since most of these fluoresce when excited by UV radiation.
Differential absorption lidar (DIAL) is the most frequently used technique for the detection of chemical warfare agents along with detection of toxic gases and pollutants. It uses two wavelengths: one corresponds to the wavelength of peak absorption of the targeted molecule, and the other to the weak absorption of the targeted molecule. Ratio of the two received backscattered signals measures the concentration of the targeted chemical warfare agent.
Fig. 3 shows the block schematic arrangement of DIAL system. Two laser pulses—called on and off wavelengths—are transmitted towards the targeted area of interest in the atmosphere, which is suspected to be contaminated with a potentially-harmful chemical warfare agent. On wavelength corresponds to the peak absorption of suspected harmful species, while off wavelength is slightly detuned from on wavelength to encounter significantly lower absorption from the same species.
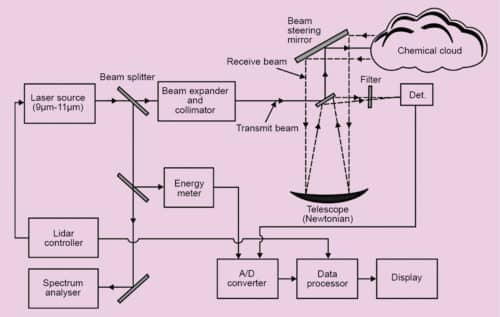
The choice between on and off wavelengths is unique for a given chemical species. It is determined by using a tunable laser that scans the area of interest by transmitting pairs of these wavelengths.
On wavelength, called ON, encounters maximum absorption when the corresponding backscattered signal is relatively weak as compared to OFF, which encounters weak absorption and produces a relatively stronger backscattered signal. This forms the basis of knowing atmospheric constituents at that time. Ratio of the two backscattered signals gives concentration of the molecules of interest.
Many chemical warfare agents have significant absorption bands in 3µm – 4µm and 9µm – 11µm bands. Carbon dioxide (CO2) lasers emitting in the latter band have been commonly used for the detection of majority of chemical agents. Some DIAL systems use both these bands and therefore employ both tunable mid-IR as well as CO2 laser sources. The schematic arrangement of DIAL system shown in Fig. 3, however, makes use of only CO2 laser.
The transmit laser beam, after suitable collimation, is directed towards the atmosphere in the desired direction with the help of a scanning gimbal mirror. The backscattered signal is received by the receiving telescope and is further focused on to the detector sub-system.
Interference filter is used to block undesired radiation. It allows only the radiation of wavelength of interest to pass through it. Detected signal is then digitised and fed to the data processor to extract the desired information on the type and concentration of chemical species.
Most information on lidar complexes specifically designed for detection and identification of chemical warfare agents is classified and not available on public domains. There are many lidar systems available for monitoring atmospheric pollutants and analysing meteorological parameters. There are some systems that are also capable of stand-off detection of chemical warfare agents such as organophosphates.
One such mobile lidar complex from Laser Systems, Russia is shown in Fig. 4. The lidar complex combines in itself aerosol lidar, wind lidar, long wave DIAL lidar and short-wave DIAL lidar, with maximum detection ranges of 20km, 5km, 5km and 3km, respectively. The system can detect hydrocarbons, Freon gases, organophosphates and so on. The scanning lidar offers azimuth field-of-view of ±180° and elevation field-of-view of –15° to +90°.
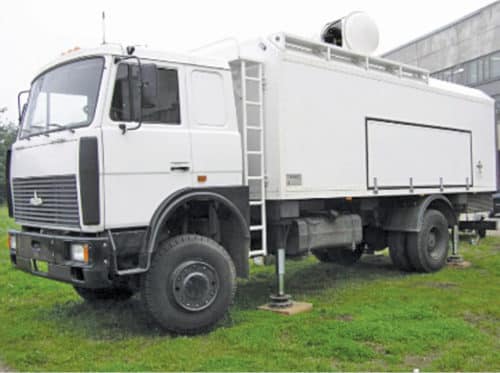
Detection of biological warfare agents
UV LIF lidar
There were several factors during 1995 – 2005 that led to increased international concern about biological warfare agents. This was the result of coordinated attacks in Tokyo using sarin gas in 1995, suspicions of an Iraqi biological warfare programme, and anthrax attacks on US Congress members and media outlets in 2001. Devastating effects of biological warfare and the ease with which a biological agent attack can be executed was amply evident from 2001 anthrax attacks in the US.
While the technologies needed for detection and identification of chemical warfare agents are more mature, stand-off detection of biological warfare agents and their discrimination from background aerosols is extremely challenging. This is primarily due to the distinction between innocuous ambient bacteria and virulent microbes, which amounts to subtle differences in their molecular make up.
As these subtle differences involve a very small percentage of molecules, these produce a very small change in their optical signatures. This makes it extremely difficult to reliably detect and discriminate between these potentially-dangerous molecules.
Processes of detection and discrimination are further complicated due to constant variation in growth media. Contaminants associated with the processing of biological warfare agents affect their optical signatures.
Stand-off detection of biological warfare agents is based on LIF effect. It is the emission from atoms or molecules after these have been excited to go to higher energy levels by excitation by another laser. Emission takes place at a wavelength that is higher than the wavelength of the laser light exciting it.
Biological warfare agent molecules mainly constitute aromatic amino acids and coenzymes. Aromatic amino acids such as tryptophan, tyrosine and phenylanine absorb laser radiation at 280nm – 290nm and fluoresce in 300nm – 400nm bands. It is therefore possible to detect biological warfare agents by using excitation by UV laser of suitable wavelength.
Also, discrimination of biological agents can be achieved only from LIF signal, because fluorescence cross-section for particles in the range of 1µm to 10µm size are sufficiently large to make single particle interrogation feasible.
Fig. 5 shows the block schematic arrangement of a typical monostatic UV LIF lidar system. Principle components of this system include frequency tripled or quadrupled Nd: YAG laser [respectively emitting at 355nm (frequency tripled output) and 266nm (frequency quadrupled output)], telescope for both transmission and reception, photomultiplier tube (PMT) for recording backscattered and bio-fluorescence signals, spectrograph with gated ICCD array for recording dispersed fluorescence spectra and lidar controller.
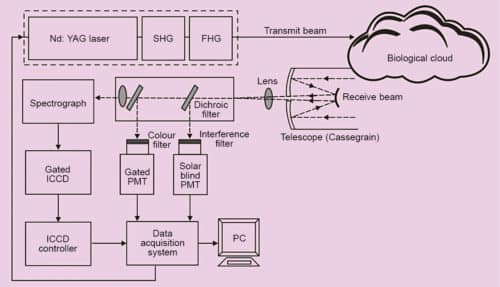
Fourth harmonic at 266nm is transmitted towards the biological cloud. The telescope receives backscattered and bio-fluorescence signals, which are then fed to PMT channel. Backscattered signal is received by gated PMT channel, and is present whenever there is a cloud along the beam path. This channel is used to measure the distance to the cloud.
Solar blind PMT channel receives fluorescence signal, and is activated only when there is a suspicious cloud. Spectrograph with gated ICCD identifies nature of the bio-molecule responsible for fluorescence. Bandwidth of the receiver channel is usually kept smaller to disallow unwanted background radiation from entering the channel.
Most information on development of systems for stand-off detection of harmful bio-aerosols such as anthrax is classified. Some experimental systems are reported in public domain. One such system is UV LIF lidar system of Norwegian Defence Research Establishment (Fig. 6).
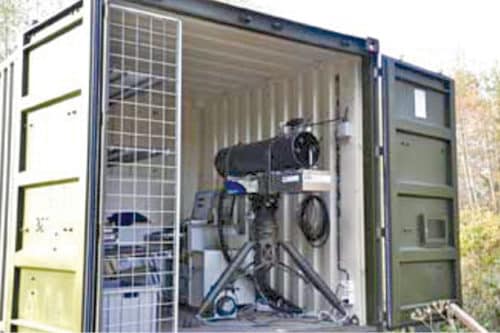
Fig. 7 shows a simplified schematic of Norwegian biological lidar. Early UV lidar systems used excitation at near 350nm. This wavelength had a fundamental problem. Many innocuous fluorophores, amino acids and other substances are also excited at that wavelength, making discrimination of threats challenging. Use of wavelength in 280nm region along with 350nm region enabled more effective weeding out of harmless targets and better identification of harmful bio-aerosols.
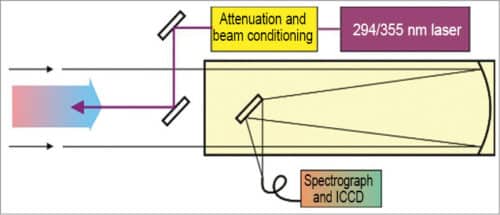
Another biological lidar system is the long-range biological stand-off detection system (LR BSDS). Shown in Fig. 8, it is used to detect, track and map large area aerosol clouds at ranges up to 30km. The system has three major components, including 100Hz operationally eye-safe laser transmitter, 61cm (24-inch) receiving telescope and transferred electron-intensified photo diode detector with an information processor integrated into a frame.
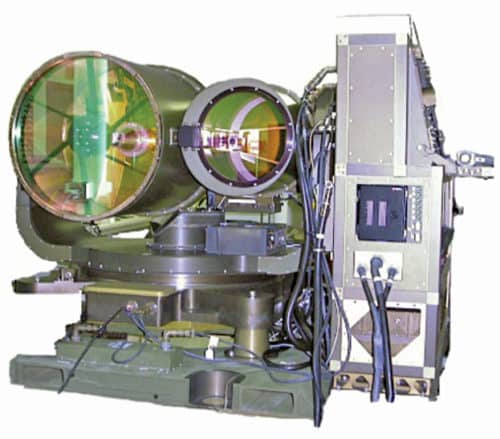
This system provides information about cloud configuration, such as size, shape, and relative intensity and cloud location including range, width, height, height above ground and drift rate. It derives power from UH-60 helicopter platform.
LR BSDS is intended to be a corps-level asset that provides early warning and information on the location and configuration of aerosol cloud formation. This information is used to enhance contamination avoidance efforts and cue other biological detection measures. Information from LR BSDS is analysed with other battlefield information and intelligence data to determine appropriate defensive measures.
Laser-based detection of explosive agents
Stand-off detection of explosive agents using laser-based trace detection methods is one of the most widely researched technologies internationally. For the technology to mature to an extent where it can be transformed into a product usable in the kind of environment or field condition usually encountered in homeland security-related applications is a great technological challenge.
One of the major problems comes from decrease in intensity of backscattered light signal due to wavelength-dependent absorption and scattering losses, and intensity decreasing inversely with distance squared. The problem is compounded by the fact that trace levels associated with common explosive agents are extremely low (in the range of fraction of parts per billion to few parts per million) for common explosives.
Another major problem pertains to unique identification of targeted explosive agents in a background of interferents. Many chemical agents have atomic compositions including sulphur, phosphorus, fluorine and chlorine, in addition to nitrogen, oxygen, hydrogen and carbon present in organic molecules. Thus, detection methodology needs to be highly sensitive and selective. Laser-based spectrometric methods have the potential of being fast, sensitive and selective with the ability to detect and identify a wide range of explosive agents, and to upgrade to handle new threats.
Atmospheric transmission at wavelengths concerned is an important factor while assessing suitability of a given stand-off detection methodology. Commonly-used technologies are laser-induced breakdown spectroscopy (LIBS), Raman spectroscopy and its variants, LIF spectroscopy and IR spectroscopy. These are all trace-detection methods; although, bulk-detection methods such as millimetre-wave imaging and terahertz spectroscopy exist.
LIBS
This focuses a high-energy laser beam on the trace sample to break down a small part of the sample into plasma of excited ions and atoms. Plasma emits light that is characteristic of emissions from ionic, atomic and small molecular species. These light emissions are detected by a spectrometer to identify the elemental composition. Fig. 9 shows a typical LIBS setup.
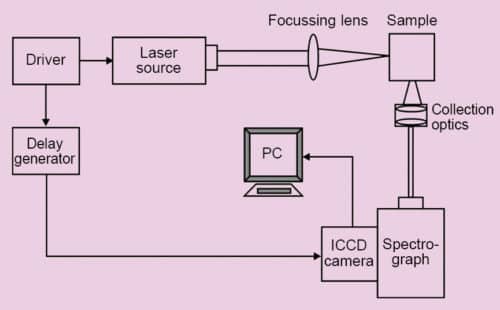
One of the challenges in using LIBS is the need to assess its efficacy to detect and identify explosive species in real environment, which is replete with many interfering substances. One way to get the desired selectivity is to use double-pulse LIBS. In this, first pulse creates the so-called laser-generated vacuum. The second pulse transmitted a few microseconds later generates the return signal. Double-pulse LIBS also improves sensitivity, in addition to enhancing selectivity. Selectivity can be further improved by adding temporal resolution to LIBS emission analysis.
Wavelength of 1064nm has been widely employed for LIBS systems. Due to serious eye hazards posed by 1064nm, scientists have tried 266nm. This also allows the designer to build Raman capability into the system. 266nm has 600 times higher maximum permissible exposure limit as compared to 1064nm.
Lawrence Berkeley National Laboratory of Department of Energy in the US has done pioneering work in developing laser ablation technologies such as LIBS for the detection of explosives and other hazardous chemicals. Applied Spectra founded by one of the scientists of Berkeley lab developed a portable LIBS-based explosive detection system.
A military prototype field version of the system (Fig. 10) was reportedly field tested on more than 100 samples in 2008. The detector was able to discriminate with 85 per cent accuracy whether tested samples contained residues of several types of explosives from stand-off distances between 30 to 50 metres, or whether composition was of materials such as rock, wood, metal and plastics.
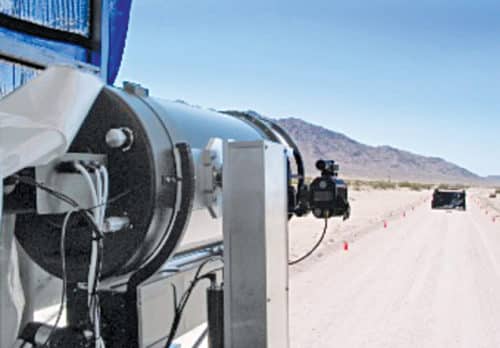
Raman spectroscopy
This offers another method for stand-off detection of explosive agents. It has been extensively used for many years as a standard analytical tool for the identification of chemical agents in the laboratory environment. Basis of detection in this case is the shift in wavelength caused by inelastic Raman scattering by the target molecule. Inelastic scattering of impinging photons where some energy is lost to (or gained from) the target molecule returns scattered light with a higher (or lower) wavelength depending upon whether energy was lost to (or gained from) the target molecule.
The difference is dictated by the energy of vibrational modes of the target molecule and therefore constitutes the fingerprint or basis of identification. Complex mixtures are identified using algorithms for pattern recognition. Fig. 11 shows a typical Raman spectroscopy setup.
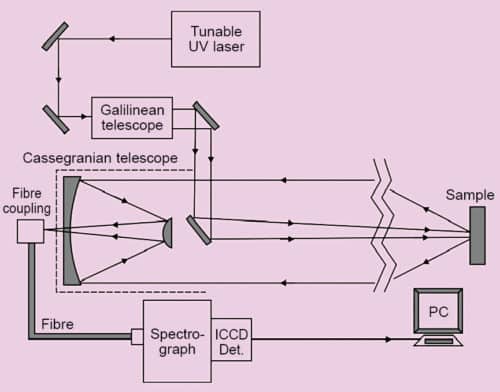
P.Eye (Fig. 12) developed by Swedish company Portendo in agreement with BAE is a handheld Raman explosives detector that can be used for the detection of roadside bombs and IEDs.
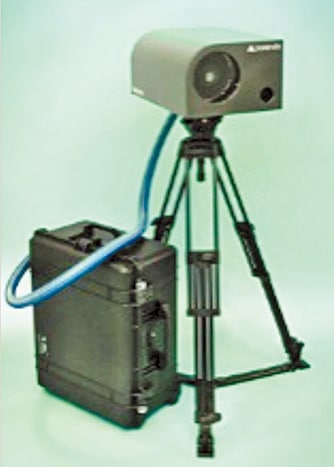
ObserveR of SciAps Inc. (Fig. 13) is another portable Raman system designed for stand-off detection and identification of explosives, toxic industrial chemicals (TICs) and toxic industrial materials (TIMs) from distances ranging from 0.1m to 3m. It has an onboard library of Raman signatures of a large number of explosive samples, TICs and TIMs, and a provision for new user-programmable entries.
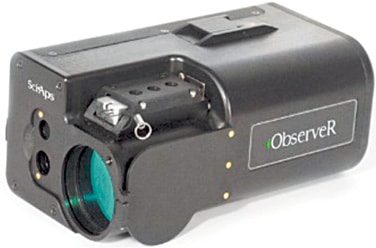
Inspector-300 (Fig. 14) and Inspector-500 are other portable Raman detectors, respectively, operating at 785nm and 1030nm, from the same company. These are particularly suited for the detection of samples that produce high levels of fluorescence.
ReporteR (Fig. 15) is a handheld Raman system from SciAps Inc. It is a miniaturised version of Inspector-300 system.
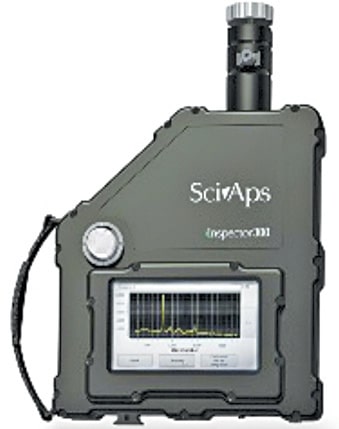
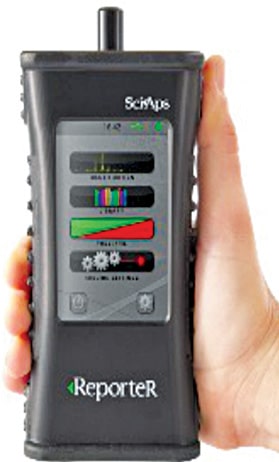
Advantage series bench-top Raman systems from SciAps Inc. offers four variants operating at 532nm, 633nm, 785nm and 1064nm.
THOR-1064 (Fig. 16) of Chemring Detection Systems is another Raman-based handheld explosive detection system. It employs a 500mW laser operating at 1064nm. It illuminates an unknown sample with laser radiation. Return signal is digitised, and Raman signature unique to the sample is recorded. An onboard processor compares the sample’s Raman signature to a stored library of Raman spectra of known compounds. A correlation algorithm identifies the best match and displays it on the operator’s console. Operation at 1064nm offers considerable fluorescence reduction over 785nm wavelength systems.
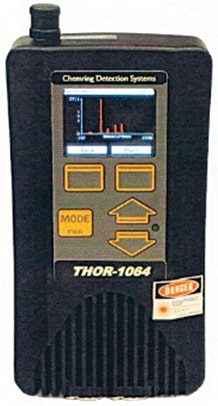
Laser Science and Technology Centre (LASTEC) of Defence Research and Development Organization (DRDO) has developed a portable Raman-based explosive detector. The system named Preemptor (Fig. 17) operates at 532nm. It can detect and identify explosive samples in solid and liquid forms from stand-off distances of up to 5m.
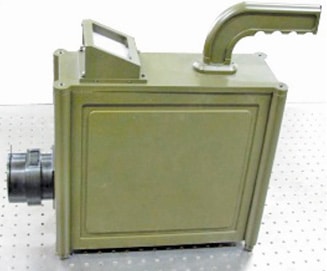
The system has potential applications in screening of liquid explosives in transparent bottles and explosive threats in the form of powder and pills. The system has been evaluated against a large number of common explosive samples, including DNT, TNT, TATP, RDX and HMX. Reportedly, the system has undergone user evaluation with paramilitary forces.
LIF is another important tool for similar applications. Though a valuable tool in combustion diagnostics and studying decomposition of explosives, it has not been found useful for the detection of explosive agents.
Almost all laser-based stand-off detection methods have detection limits too high to make these suitable for the detection of explosives in field conditions. Such detection requires the capability to detect traces in vapour phase or in the form of particles.
Another problem is insufficient selectivity to identify target explosives in the presence of interferents. In fact, none of the laser-based spectroscopic methodologies available today are ready for full-functionality prototype manufacturing.
There is lot of scope for further research to improve the performance of more mature technologies in terms of both detection sensitivity and selectivity. The final solution would probably lie not in one type of sensor, but in the integration of several technologies to derive the benefits of the best of each. Simultaneous use of stand-off LIBS and stand-off Raman has been exploited successfully.
To be concluded…
Dr Anil Kumar Maini was formerly outstanding scientist and director at Laser Science and Technology Centre (DRDO)
Nakul Maini is post graduate in optical engineering from University of Bristol (UK), currently working as senior engineer (product development) at Tek Xplore, New Delhi